The first functionally vascularized synthetic hydrogel
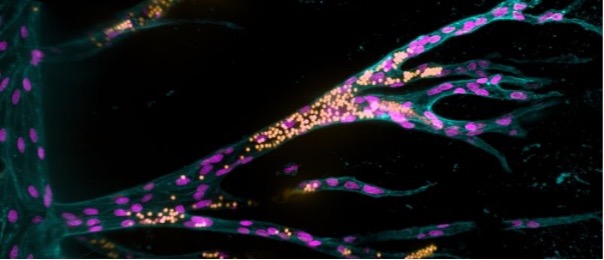
In a world-first, researchers have developed a synthetic hydrogel scaffold that can be functionally vascularized.
To bioengineer functional tissue to repair damaged organs, a multitude of factors need to be considered. One aspect currently presenting bioengineers with a particular challenge, is the stimulation of angiogenesis in these replacement tissues in order to form functional blood vessels.
Some studies have managed to achieve the feat using hydrogel scaffolds formed with naturally-occurring extracellular matrix (ECM) proteins, but these scaffolds lack the structural integrity to be utilized in vivo. Conversely, more robust synthetic scaffolds have proven difficult to vascularize.
A team of researchers from the Max Planck Institute (Munster, Germany), led by Britta Trappmann, has set out to identify the conditions required to induce successful vascularization in synthetic scaffolds. By upgrading an existing microfluidic in vitro model, developed during Trappmann’s time at Harvard University (MA, USA), the team were able to successfully stimulate the growth of a functional blood vessel system in a synthetic hydrogel.
The initial model consisted of a 3D sugar-based hydrogel with two parallel 400 μm diameter holes, 1 mm apart, installed using acupuncture needles. The team seeded the first channel with endothelial cells, which formed a blood vessel after 1 day. Once the ‘parent’ blood vessel is formed, the second hole can be filled with a solution of growth factors that stimulate angiogenesis, promoting the migration of endothelial cells into the hydrogel.
Exploring the regenerative potential of the inner ear
The hearing organ in the inner ear, called the cochlea, contains two types of sensory cells: ‘hair cells’ that have hair-like cellular projections that receive sound vibrations; and ‘supporting cells’ that play vital structural and functional roles.
To identify the hydrogel properties that most successfully enable the formation of blood vessels from these migrating cells, the team altered the composition of the hydrogel, varying the concentrations of the cell-adhesive protein RDG. RDG was selected for its ability to activate integrins – adhesion molecules in the membrane of endothelial cells that enable them to attach to their surrounding environment.
Increasing concentrations of RDG led to an increase in endothelial cells migrating together, as opposed to individually, making it possible for them to form tubular structures. The team were also able to identify that the integrin essential to this co-migration was αvβ3. While tubular structures of vascular cells did form, initially they were too small to be considered truly functional, in part due to the stability of the scaffold.
To correct this, the team incorporated crosslinker molecules between the sugars of the hydrogel that were more easily cleaved by molecules released from the endothelial cells, like matrix-metalloproteases. By increasing the ‘degradability’ of the hydrogel, the cells were able to migrate faster and form larger vascular structures, successfully installing a functional vasculature into a synthetic hydrogel.
While the team have succeeded in this regard, the long-term goal of this project is to collect enough information to create fully functional implantable tissues; a goal that will require a whole host of other cell types and biomolecules to be incorporated. “Moreover, the effect of all these factors is linked in natural tissues and varies from organ to organ,” noted Trappmann.
There is plenty of work to be done, but for now one of the great conundrums in tissue engineering has been put to bed.