The spark of life
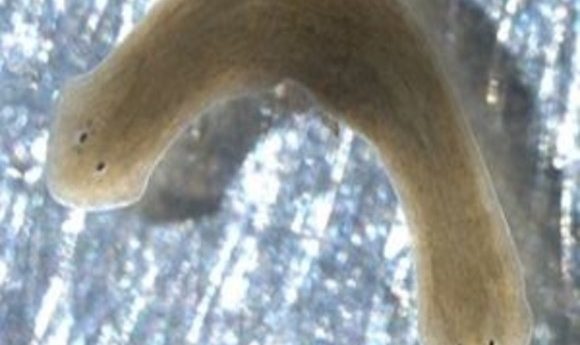
Electrical signaling isn’t unique to neurons; cells across the body use voltage gradients in development and other processes. Researchers are getting closer to solving the riddle of bioelectricity.
Under certain conditions, planarians can be made to “remember” and repeatedly regenerate a two-headed body plan.
Credit: Nogi, T., et al. “A novel biological activity of praziquantel requiring voltage-operate Ca2+ channel beta subunits: Subversion of flatworm regenerative polarity.” PLoS Negl Trop Dis 3.6 (2009): e464.
Which direction does a two-headed planarian glide?
Actually, that’s not a big question, said Michael Levin of Tufts University in Medford, Massachusetts (USA). “Sometimes they do get confused, and sort of go in opposite directions,” he said, but most of the time the two heads, one on each end of the flatworm, cooperate. “They’re perfectly viable.”
Levin and his group use two-headed planarians, among other model systems, to study the role of electricity in biology. Bioelectricity, to Levin, means the voltage changes, ion currents, and electrical fields that are essential for embryonic development, cellular metabolism, gene expression, and many other processes. While researchers have known about bioelectricity beyond the nervous system for upwards of eight decades, most scientists were distracted by the rise of molecular biology in the late 20th century, leaving this field behind. Levin’s research, along with his efforts to spread the word about bioelectricity, are helping to bring about a modern renaissance of interest in the field, noted Dany Spencer Adams , a developmental biologist at Tufts working on electricity and facial development [1], and a former postdoctoral fellow in the Levin lab.
There’s good reason to pay attention. For example, mutations in genes for ion transport channels can lead to birth defects, such as Andersen–Tawil syndrome [2]. Many medications that target ion channels—for example, drugs for epilepsy or psychological disorders—are dangerous during pregnancy because they alter the embryo’s electrical patterns. Bioelectricity is key in the regeneration of limbs by amphibians [3], and might someday figure into regeneration treatments for people. What’s more, as they transform, cancer cells tend to isolate themselves from the surrounding bioelectric milieu, suggesting that reversing that process might prevent tumors or normalize the tissue therein, Levin suggested.
“In the same way that biochemistry kind of underpins everything in biology, I think that bioelectricity is a parallel source of understanding,” said Adams. “It’s just as fundamental.” Adams is editor-in-chief of a new journal, Bioelectricity, set to launch fully in 2018.
It’s hard to fault scientists for turning to the molecules of DNA, RNA, and protein; they’re easier to study, especially in dead or fixed tissues. Bioelectricity is different: “The minute the cell dies, it’s gone,” said Levin. His lab is the first to develop and apply modern tools for joining bioelectricity to molecular biology. For example, voltage-sensitive fluorescent dyes help researchers map the electric fields across an organism. “You can literally see all the electrical conversations that cells are having with each other,” he explained.
They can also make changes to an organism’s bioelectric patterns by altering the expression of ion channels or treating organisms with neurotransmitters or drugs that affect them. Up-and-coming tools also add optogenetics, either to observe or alter electrical fields in vivo.
Are two heads better than one?
Levin’s graduate student Fallon Durant recently published their research on the two-headed flatworms [4]. Planaria reproduce by fission, splitting into two pieces that each regrows the rest of its body [5]. How does one piece of the organism arrange the rest?
Durant predicted that it comes down to bioelectric patterning. To disrupt the planarian’s electric networks, she treated them with octanol. This shuts down cell-to-cell communications via gap junctions, which normally can transmit information electrically. When Durant cut planarians into pieces in octanol, those pieces regenerated flatworms with two heads, instead of one head and one tail.
“What it means is, the pattern to which this animal regenerates upon damage is labile,” explained Levin. Moreover, the worms’ descendants ‘remembered’ that new body plan and continued to spawn two-headed progeny, even months after the octanol was gone.
To observe changes to the planarians’ bioelectric patterns, Durant soaked them in a fluorescent voltage reporter. In one-headed flatworms that were destined to generate single-headed offspring, the voltage formed a gradient, more depolarized in the head and more polarized in the tail. But in those that would go on to make two-headed wormlets, the tail was depolarized too.
Could Durant change that abnormal bioelectric pattern back to normal? She treated the double-headed flatworms with a drug that promotes polarization of planarian tissues. Sure enough, many of the offspring reverted to the one-head body plan.
This type of “memory” isn’t limited to flatworms; a similar kind of malleable morphological programming seems to exist in the regeneration of deer antler point patterns and the left- or right-handedness of fiddler crab pincers [6,7]. Regenerative medicine might take advantage of these bioelectric systems, for example by using ion-transporting drugs—‘electroceuticals’—already approved for human use, Durant and Levin suggested.
Brains and beyond
Researchers in Levin’s group also work with embryos of the frog Xenopus laevis, where they’ve discovered that the brain both depends on, and generates, bioelectric signals needed for proper development. Early in the process of building the tadpole’s brain, cells lining the neural tube are hyperpolarized, while those in the surrounding area are depolarized. When postdoctoral fellow Vaibhav Pai disrupted this patterning in the neural tube, he created embryos with terrible brain defects, including a lack of nostrils, olfactory bulbs, and forebrains. When he allowed the hyperpolarization to spread beyond the spot where the brain belonged, it created neural-like tissue elsewhere in the embryo. Pai also found that when he caused brain and bioelectricity defects with drugs, or by over-activating the Notch pathway, he could rescue the brain by restoring the proper voltages [8].
Not only does the brain depend on bioelectric fields, it generates electrical patterns needed elsewhere in the body, discovered postdoc Celia Herrera-Rincon. When she removed early brains from frog embryos, muscles and peripheral nerves developed abnormally. However, overexpression of an ion channel rescued the muscles, suggesting there was a voltage patterning deficiency when the brain was missing [9].
For now, bioelectricity remains a small field of study. However, every time a scientist studies a disease of ion channels, or changes to body structure, or electrical effects in vitro, bioelectricity is there, Levin pointed out.
“It’s bigger than people think it is,” he said. “There’s so much to do.”