Has the mystery behind a common cancer mutation been solved?
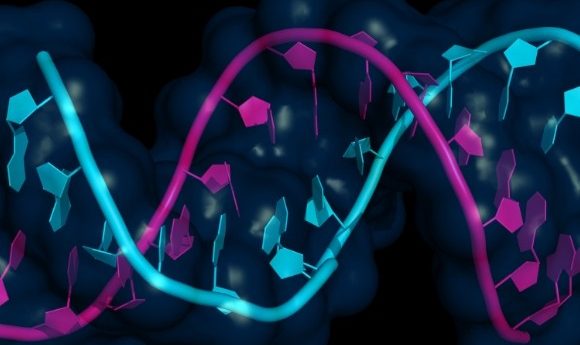
Researchers have shed light on the mechanisms behind a previously poorly understood cancer mutation.
A collaborative study led by Robert Bradley (Fred Hutchinson Cancer Research Center; WA, USA) and Omar Abdel-Wahab (Memorial Sloan Kettering Cancer Center; NY, USA) has discovered how mutations in the SF3B1 gene, which codes for the SF3B1 splicing factor, contribute to the formation of many common cancers.
SF3B1 is an RNA splicing factor and has been implicated in several important spliceosome processes. The spliceosome is responsible for removing so-called ‘junk DNA’ during transcription. When the spliceosome is impeded it has a detrimental effect on the translation of proteins, resulting in vital proteins not being produced or being malformed.
“We know many of the genetic mutations that cause cancer and that mutations in SF3B1, in particular, are strongly associated with many cancer types,” explained Bradley. “What’s been unclear is why mutations in SF3B1 are so common and how to best identify treatment options. Thanks to breakthroughs in sequencing technology, computing power and CRISPR genome engineering, we were able to discover how SF3B1 mutations cause cancer and potentially block the process of tumor progression.”
The team analyzed SF3B1 mutations in several different cancer types and identified 19 common mutations. Positive-enrichment CRISPR screens were utilized to recreate every mutated variant. These screens demonstrated that every mutation in SF3B1 had the same knock-on effect on the expression of the gene BRD9. The resulting disruption to the BRD9 protein drove tumorigenesis.
- HPV-RNA Seq: a powerful diagnostic technique for cervical cancer?
- Boosting natural killer cells could help fight cancer
- Could photothermal therapy become the ‘gold standard’ of cancer treatment?
Further screens demonstrated that SF3B1 affects the transcription of BRD9 consistently, no matter the mutation. The SF3B1 protein fails to remove ancient viral RNA when transcribing BRD9. The inclusion of the viral element means that BRD9 RNA is not recognized by cellular machinery and is subsequently degraded.
The opportunity to intervene in the transcription of a singular protein offered a unique therapeutic opportunity. Bradley and Abdel-Wahab utilized a combination of CRISPR-directed mutagenesis and antisense oligonucleotides to correct the mis-splicing of BRD9.
“It’s striking to me that we could correct splicing of a single gene and slow tumor growth,” Bradley commented. “Since hundreds of genes have errors in splicing in these cancers, I’d think it would be probably equally likely that you’d have to correct splicing of 50 of them to have any effect on the tumor. In fact, you can just hit one.”
The strategy was tested in mice implanted with human melanomas. It was demonstrated that by preventing the inclusion of the unwanted viral RNA when BRD9 is transcribed, the accurate assembly of the BRD9 protein was ensured. When BRD9 functioned correctly, the growth of the melanoma tumors slowed.
While these results increase our understanding of the genetic interactions within common cancers, targeting SF3B1 mutations is a long way from being a therapeutic possibility in humans. Bradley and Abdel-Wahab now hope to collaborate with experts to gather more information and develop their early findings into a real treatment strategy.