Clap on, clap off. Protein on, protein off
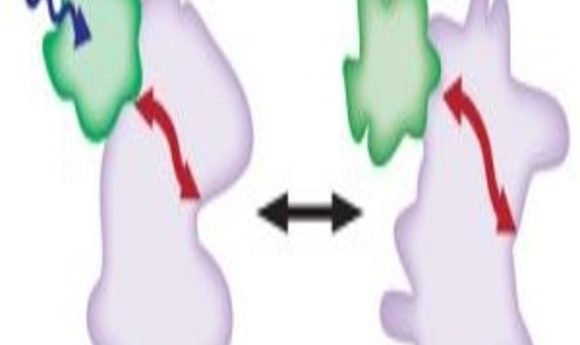
New light-controlled proteins may improve our understanding of cell structure, function, and behavior.
Protein inhibition using light. Light (blue arrow) activates the LOV2 domain (green) which causes the protein of interest to undergo a conformation change (pink/red arrow).
Image courtesy of Onur Dagliyan.
In the dark, the 2 Dronpa domains bind together (green) and block the active site of the kinase (blue). When light shines on the protein, the domains dissociate (gray) and uncover the kinase active site.
Image courtesy of Michael Lin.
In the game telephone, players whisper a phrase from one ear to another through a chain of people. As the message travels, the words are often misheard, distorting the original phrase. Cells use signaling pathways in a similar fashion, where proteins relay messages to direct the cell’s actions. However, this message must remain clear so that cells function appropriately.
Studying the dynamic behavior of cells requires manipulating the proteins involved in signaling pathways and watching what happens. Although traditional techniques using drugs or genetics to manipulate proteins are readily available and straightforward to use, drugs lack specificity and genetic manipulations can take a long time, allowing the cell to compensate in other ways.
“We need a fast, reversible, and non-invasive way to control protein activity. We decided to use light,” said Onur Dagliyan, from Harvard University.
Unlike genetic or pharmacological manipulation, light-controlled proteins respond immediately, can be temporally and spatially triggered, are reversible, and are specific to the protein of interest. However, designing and using light-controllable proteins often requires expertise and specialized equipment. Now, two recently published articles in Science describe generalizable methods for making photo-controllable proteins (1,2).
Light On: Protein Disorder
In 2010, Klaus Hahn and his team at the University of North Carolina at Chapel Hill, set out to develop a method to control protein activity by placing a “protein knob” at a precise site on a protein of interest so they could turn the protein off using the drug rapamycin. However, while conducting a control experiment, the team was disappointed to find that attaching the protein knob killed protein activity without the addition of rapamycin. Adding rapamycin actually restored protein activity. The same unexpected phenomenon worked for several different proteins (3).
After joining Hahn’s group in 2011, Dagliyan determined how rapamycin activated proteins with the attached knob (4). “Mother nature already has a great way to control proteins. There are thousands of proteins in the cell, they are all bumping each other and so on. They have to be active and inactive at certain times and places, right? And usually they do that through conformational changes,” said Dagliyan. Using molecular dynamics simulations, Dagliyan found that adding rapamycin to the knob-controlled protein caused the protein to transition from a disordered, or inactive, to ordered, or active, state. In other words, the knob caused the protein to undergo a conformational change.
After determining the mechanism for protein inhibition using rapamycin, Dagliyan applied the same technique but used light to inhibit protein activity by attaching the light sensitive protein domain, LOV2, to a protein of interest (1).
“We were working with [LOV2] for other purposes. It begins very tight, but when you irradiate it, it becomes very loose. So what if we put that in the same exact site and see if we have the opposite effect? Instead of activation by rapamycin, we would have inhibition by light,” said Hahn. Indeed, shining light on a protein with a precisely attached LOV2 domain induced protein disorder.
“Once we realized [the mechanism], it was easy to apply to other proteins,” said Dagliyan. The researchers generalized their technique to control proteins with light by attaching the LOV2 domain to loops on the outside of a protein and connected to the active site and generated several types of proteins, including kinases and phosphatases, involved in cell movement.
“Here the cell doesn’t have time to change. It doesn’t know what hit it until you turn on the light. So that’s what makes it really cool,” said Hahn. Since the method developed by Dagliyan and Hahn uses light to cause protein disorder, which can either inhibit or activate the protein, the cell functions normally in the dark. Protein conformational changes controlled by light may be advantageous in some assays, but may not be applicable to all biological questions. So, what other methods can you use to control proteins with light?
Light On: Protein Uncaging
Michael Lin at Stanford University and his team designed a general way to activate kinases using light (2). Lin reasoned that using two protein domains that bind together in the dark and come apart in the light could control protein activity. By strategically attaching the domains on either side of the kinase active site, they could essentially cage, or turn off, the protein in the dark and uncage it in the light. “I thought that was the simplest way to do it,” said Lin.
Since no naturally occurring proteins dissociate with light, Lin turned to his favorite green fluorescent protein Dronpa which he had made into a tetramer back in 2012 (5). “I realized we could engineer Dronpa so that it was dimeric in the dark but would be monomeric upon illumination,” said Lin.
Lin shared the idea with first author Xin Zhou, then an undergraduate student in his lab. Zhou taught herself how to clone and within three weeks presented Lin with exciting results. “It’s not every day when you think of an idea that’s never been tried before and the very first experiment shows that it works!” said Lin.
Lin and his team first applied their technique to kinases with similar 3-dimensional structures and validated that they were able to activate kinases with light using a fluorescent biosensor. After confirming that their technique worked for kinases with similar structures, they moved to kinases with varying structures to ensure that their methodology was generalizable. Using light and the modified Dronpa, which they named pdDronpa1, Lin and Zhou turned a variety of kinases on and off over several rounds.
By combining the light-controlled kinase with a fluorescent biosensor, they developed an all-optical method to screen proposed drugs that inhibit kinases. Additionally, Lin and the team turned kinases on and off in vivo in C.elegans, demonstrating that their technique can be used both in cell culture and live animals.
As with any technique, pdDronpa1 has advantages and disadvantages. On one hand, a fluorescent protein provides a useful readout concerning whether the protein is active or inactive. Additionally, pdDronpa1 activation requires a longer wavelength of light, making it less phototoxic than other photo-controllable proteins. However, activation requires a large amount of light, which Lin hopes to improve in future iterations. Despite this limitation, Lin’s design can be used for several different kinases and provides an additional method in the toolbox to study signaling mechanisms.
Technology to Help Biology
“We develop technology because we want to help scientists do biology,” said Lin.
“More importantly, this will become a generally applicable tool where the rules that [we] came up with are good enough that people really can start doing this for other kinds of proteins. That would be wonderful,” said Hahn.
Although both techniques use light to control protein activity, they each take advantage of different protein properties to reach their goal. Dagliyan and Hahn use light to inhibit or activate protein activity by inducing a conformational change, while Lin uses light to activate proteins by caging and uncaging the protein active site. Both tools can answer fundamental biological questions.
“We can manipulate very specific things in exact places and exact times and even study behaviors that happen over seconds,” said Hahn. It is no doubt that optically controlled proteins will provide fundamental insights into the signaling pathways that control dynamic cellular behavior.