Making proteins the spider silk way
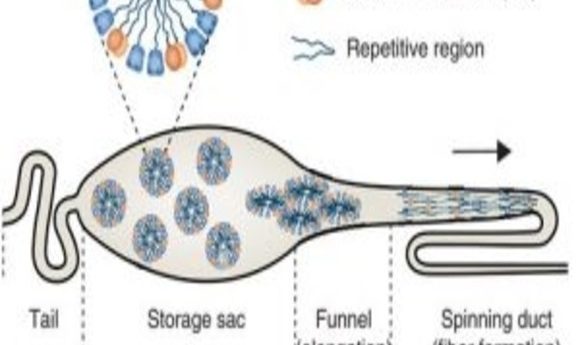
A small fragment of a protein from spider silk enables researchers to express functional hydrophobic and aggregation-prone proteins.
As scientists have learned more about the structure and synthesis of spider silk, there has been a growing interest in the unique properties of spidroins, the main proteins found in spider silk fibers. For example, these proteins form fibers that are incredibly strong and elastic, as well as extremely biocompatible, prompting efforts to produce synthetic spider silk for biomedical applications.
Now, researchers at the Karolinska Institute in Sweden have developed a new method for production and purification of hydrophobic or aggregation-prone proteins by exploiting another unusual property of spidroins.”The manufacturing process is based on the method spiders use to keep their extremely easily aggregated proteins soluble for silk-spinning,” said Professor Jan Johansson, co-lead author of a new study in Nature Communications, in a press release.
In spider silk glands, the storage sac compartment contains spidroin proteins organized into soluble micelle-like structures with the hydrophilic amino- and carboxy-terminal regions occupying the surface, while the bodies of the proteins, which contain alternating hydrophobic and hydrophilic domains, are sequestered within the micellar interior. During silk production, these proteins move out of the spinning sac to the spinning duct, following a gradient of decreasing pH. The micellar complexes are disrupted as the pH falls below 6.5, in part due to dimerization of the amino-terminal domain (NT) regions of the spidroin proteins, allowing the proteins to associate with one another during the spinning process to form highly organized spider silk fibers.
With this mechanism in mind, Johansson and his colleagues sought to use a highly conserved spidroin NT sequence as a solubility tag, theorizing that fusing this peptide to a protein of interest would prevent protein aggregation and misfolding during recombinant expression in E. coli. To enhance the solubilizing effect of NT, they created a “charge-reversed” NT mutant that does not dimerize, increasing the pH range at which a monomeric NT domain promotes organization of the fusion protein into micelle-like structures.
“We had bacteria produce this part of the protein and then linked it to different protein drug candidates,” said Anna Rising, also a co-lead author of the study. The authors tested this approach with a number of hydrophobic or aggregation-prone proteins that have been difficult to produce by recombinant expression. In all cases, NT fusions produced more soluble protein than fusions with other solubility tags currently in use.
The team then demonstrated that they could purify two of these fusion proteins, an analog to surfactant protein B and another to surfactant protein C, both of which have been used to treat or prevent respiratory distress syndrome in infants. “We chose to produce lung surfactant protein C because it is probably the world’s most aggregation-inclined protein,” said Rising.
To do this, the researchers devised a streamlined purification protocol for purifying recombinant protein without the need for time-consuming chromatography steps to remove the NT peptide. As a result, the method is inexpensive, efficient, and could easily be scaled up to produce large quantities of pure protein.
The surfactants in clinical use are generally mixtures of proteins isolated directly from animal tissues. The production of pure preparations of a wider range of surfactant protein variants could be a big boost for basic research, drug screening, and clinical application of these proteins.
“Since this production method is much simpler and cheaper, it might one day be possible to use our synthetic lung surfactant to treat more lung diseases than just preterm babies,” added Johansson. “The method will also hopefully enable the production of other biological drugs.”