Antivenom intelligence? The role of AI in developing snakebite treatments
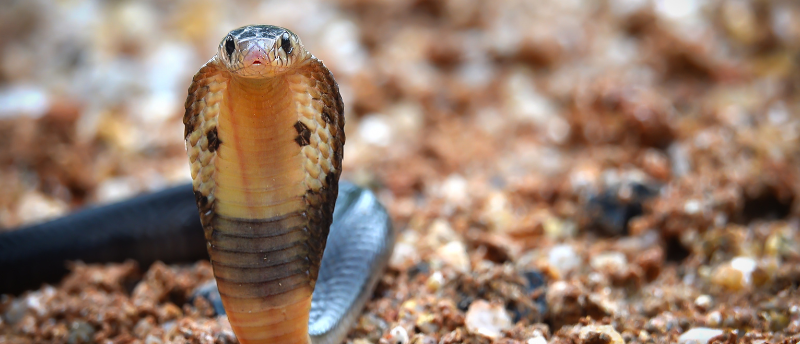
We spoke to Tim Jenkins, an Assistant Professor at the Technical University of Denmark (DTU; Copenhagen, Denmark), about his research incorporating artificial intelligence into antibody discovery programs to develop new snakebite antivenoms.
Snakebites present a neglected public health issue in many tropical and subtropical countries – an estimated 5.4 million people are bitten by snakes each year, and roughly half of those people are injected with venom. Between 81,000 and 138,000 people die as a result of snakebites, while around three times as many are left with permanent disabilities [1].
Although antivenoms exist, there are many barriers to making them safe, effective and accessible to those who need them. Current antivenoms are made following a 100-year-old method of injecting a venom of interest into a production animal, such as a horse, waiting up to a year for the animal’s immune system to generate antibodies and then collecting the blood plasma from the animal and purifying it. “It works, but it has a lot of downsides,” Tim explains. The resulting antivenom is not tailored, so it might not target the most clinically relevant toxins and can cause adverse reactions; it’s not pure, so large quantities are required for successful treatment; and the manufacturing pipeline is lengthy, and upscaling is challenging, driving up the cost. These factors make snakebite a huge socio-economic burden, impacting those in poorer, rural communities most. “It can cost a farmer in Africa more than he makes in a year to pay for just the vials of antivenom, not even the hospital treatment.”
Researching and developing new snakebite treatments that address these challenges is difficult, mainly because snakebites are almost absent from the global health agenda, meaning funding is limited. “If we are talking about treating all snakebite across the world, we have about 2000 toxins that we need to potentially neutralize. How do we figure out which ones are the most important? How can we develop more effective antibodies and make the product cost effective and affordable to those most in need?”
Same same, but different
To develop new snakebite treatments in the most cost-effective way possible, Tim and his colleague Andreas Hougaard Laustsen-Kiel, a Professor at DTU, decided to use next-generation treatments that have been developed for other more well-funded diseases, such as cancer and HIV, and apply these to snakebite.
Specifically, they are developing broadly neutralizing recombinant monoclonal antibodies utilizing in vitro antibody discovery technologies to identify specific antibodies against snake venom toxins [2]. “Phage display is our bread and butter. We established this together with one of the inventors of phage display, John McCafferty. Over the last year, we also started implementing yeast display, which is a different in vitro technology. We now combine these two technologies for their different benefits.”
Phage display may be their current bread and butter, but computational tools really take the cake.
Computer says yes
During the COVID-19 pandemic when access to labs was restricted, Tim was pushed back into the next-generation sequencing roots of his PhD and started applying computational ideas to antibody discovery. “That’s where my research group that I started about a year ago is positioned – at the nexus between lab-based in vitro science and more computational in silico aspects of science.”
In the last 6 months, his group has leaned into generative antibody design, which was sparked by recent publications from David Baker’s group at the University of Washington in Seattle (WA, USA). Baker and his excellent team have pioneered methods to predict and design protein structures and recently developed a powerful new tool that can be used to design proteins by combining structure-prediction networks and generative-diffusion models, called RoseTTAFold (RF) Diffusion [3]. The tool is incredibly effective – previous design methods required hundreds of thousands of variants to be tested before finding one that performed as desired; however, with RF Diffusion, 100 designs would be tested and 10% could be viable candidates. “That blew my mind. We reached out to the Baker Lab in early January and asked if they might be interested in collaborating. Fortunately, we were able to convince them that snakebite was a cool area to try this tool out in.”
The tool worked, and within four weeks of using it, the collaboration with Susana Vazquez Torres, a grad student at the Baker Lab, had yielded binders against toxin groups that they had been struggling with in the lab for years. “AI is now starting to really revolutionize science. It’s been on the horizon for 5–10 years now, but we haven’t really seen anything transpire. But what we have seen over the last year and what we are going to see over the next 2 or 3 years is going to change the way that biotherapeutics will be discovered, developed and manufactured.”
Hostess with the mostest: studying host–pathogen interactions
In this interview for our Spotlight on antibody discovery, we talk to Pontus Nordenfelt about the role antibody discovery plays in his research, the discoveries this has enabled and how this research can contribute to the fight against antimicrobial resistance.
What happens next?
So, what happens when these entirely in silico proteins are taken to the clinic? Although the team know that the proteins are good binders, they need to establish how immunogenic they are and if these novel structures cause any unwanted side effects that they haven’t been able to predict. Tim isn’t too worried about this, as the protein suite that these tools have learned from are all real, published proteins and should be reasonably safe to work with.
Tim is more concerned about what the landscape will look like if they go to regulatory approval. “When you are developing something for cancer, you usually have the money, so you can spend time waiting and figuring these things out. But in the field of snakebite envenoming, you have to be so cost effective that you can’t be wasting precious time and need clear regulatory guidelines for these new molecules as soon as possible.”
Money, money, money
With factors out of their control, like government approval and distribution, driving up costs, what is the team doing to ensure these antivenoms are cost effective? The main factor is developing binders that are broadly neutralizing [4], meaning that one binder can work against a range of different toxins that are still similar enough in structure to be recognized. “If you manufacture 10s of antibodies, it’s exponentially more expensive than manufacturing a handful in the same product. Driving down the number of different antibodies we need to produce in the same batch for one antivenom will drive down the cost of manufacturing, which will help make the product more affordable.”
Looking to the future
For Tim, the next challenge is harmonizing all the techniques him and his team are currently using, both in the lab and computationally, with automation. “A lot of our efforts are focused on how we miniaturize assays, scale them up, make them high throughput and automate them so we can link these powerful computational tools to the lab as effectively as possible.”
This will help achieve Tim’s dream of having a semi- or fully automated setup for designing proteins on the computer: “This would mean that for a new viral outbreak, we take the sequence of the pathogen and within a day, we could design a library of different binders against these pathogens. Within that same day, we might even be able to synthesize the DNA, express these binders and possibly test them. So, go from a pathogen sequence to a binder that can go into more sophisticated, either functional validation or maybe even animal settings within a few weeks time using automation technologies. I think that’s the direction the field has to move in and we’re already beginning to see that.”