Neuroscience: bridging the gap between cell-based and human research
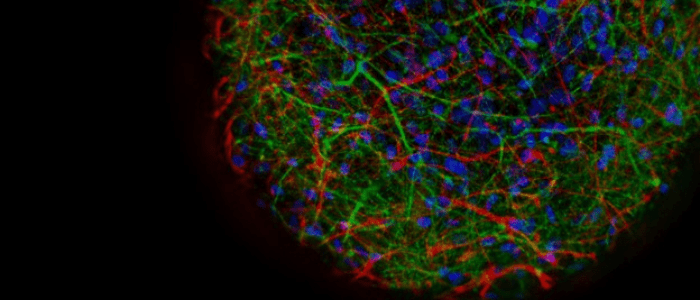
The rise of neurological disorders and dysfunction.
Neurological disorders impact up to a billion people around the world and appear to be on the rise [1]. In fact, those affecting the central and peripheral nervous systems – including stroke, migraine, Alzheimer’s and other dementias – are the leading cause of disability and the second leading cause of death worldwide [1, 2].
Heightened awareness of the challenges that accompany neurological disease prevention and treatment is driving the critical need for accelerated research to improve therapeutic intervention. By better understanding the biology and causes of these diseases at the cellular and molecular levels, we will be able to find better treatments, aid in prevention and ultimately alleviate the burden such diseases and disorders place on individuals, families and healthcare.
The increase in neurological disorder diagnoses is believed to have several potential underlying etiologies, including decreased infant mortality rates, longer life expectancies and better diagnostic capabilities. Additionally, environmental chemicals may play a role; the number of untested chemicals in the environment is increasing, which may have adverse effects on human health [3].
In 2012, scientists identified several environmental chemicals involved in widespread neurodevelopmental behavioral and cognitive issues, including ADHD, autism spectrum disorders and lower IQs [4]. Called out were substances like lead found in paint products, methylmercury present in large fish, and organophosphates common in agricultural pesticides. As subsequently covered in The Atlantic, most of the listed environmental chemicals had already been heavily restricted; however, it did reveal a massive chasm in rigorously testing chemicals for safety before adding them to common household products, such as furniture, clothing and even food [5].
Furthermore, unanticipated neurotoxicity is one of the leading causes of unwanted side effects of pharmaceutical drug development and use. It is a leading cause of attrition during development and failed clinical trials, leading to expensive delays and even preventing potentially useful drugs from reaching the intended patient population [13]. Clinically, neurotoxicity is of growing interest as many drugs that are commonly prescribed by treating physicians – like antibiotics, therapeutic drugs, anti-cancer drugs and cardiac drugs – may precipitate neurotoxic signs, such as peripheral neuropathy or confusion, or even exacerbate an underlying neurologic disease [6].
There is clearly a great need to develop methods to provide insight into the causes and effects of neurological diseases, as well as rapidly evaluate potential neurotoxic effects of environmental chemicals and new drugs. Human induced pluripotent stem cell (iPSC)-derived tissue cells in high-throughput organotypic structures combined with automated functional and structural imaging assays accurately represent human biology and provide the throughput necessary for industrialized testing. These technologies open new opportunities to employ physiologically relevant in vitro model systems to understand and develop treatments for neurological disorders, test environmental chemicals and ensure that safe and effective drugs reach the clinic.
Advanced human cell models pave the way for discovery
With current approaches for neurotoxic evaluation of chemicals relying mostly on non-human animal models, we recognized and are tackling an urgent and growing need for more complex, biologically relevant and predictive in vitro assays. For the past several decades, scientists have almost exclusively carried out biomedical research in animal models. However, in addition to the ongoing moral arguments to reduce the practice of using animals in biological experiments, there are also significant scientific limitations with animal testing [7]. Animals such as mice are complex organisms and may be susceptible to many of the same health problems as humans. However, while the significantly shorter life expectancy of mice ensures they can be studied throughout their life cycle and these testing practices have led to a deep understanding of many fundamental biological processes, recent studies have identified significant differences between such animal models and humans, bringing into question the relevance of such models [8]. It is now clear that this approach has left gaps in our understanding of human-specific developmental, cell biological, physiological and disease-related events.
With the advent of human organoid models [9], scientists can take a personalized approach to their research and study tissues in remarkable detail like never before. Using organoids that resemble human tissues, scientists can now investigate many of the morphogenic events leading to tissue formation as well as cell-to-cell interactions within the tissue avatars, which can reveal a great deal about how certain compounds affect human neurologic function.
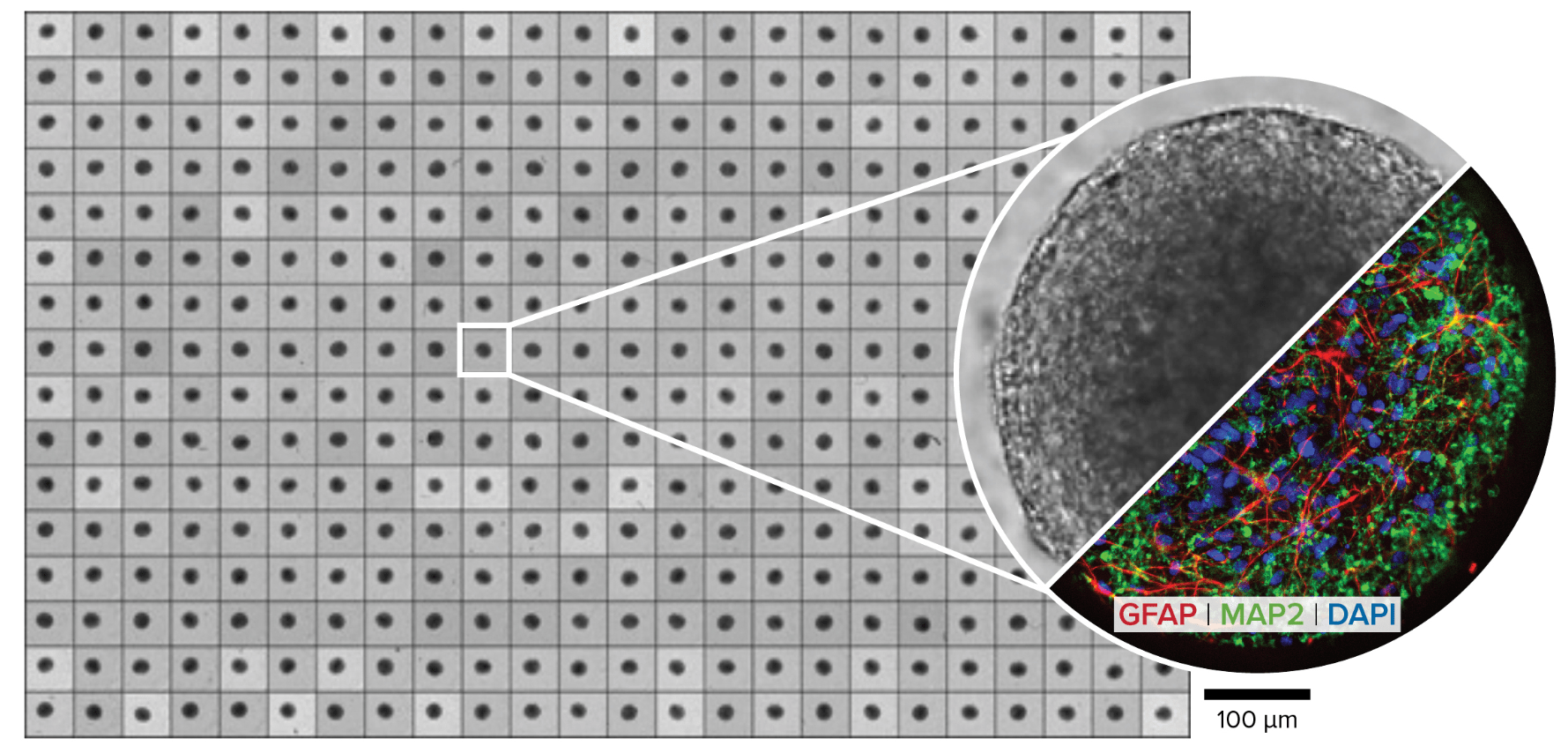
Figure 1. microBrain 3D, a robust, reproducible, high-throughput 3D human neurospheroid for drug discovery. microBrain 3D neurospheroids contain approximately equal numbers of astrocytes (GFAP) and neurons (MAP2) and are provided in 96- or 384-well plates where each well contains a single neurospheroid of consistent size.
How we advance understanding of neurological disorders
Inspired to push the boundaries of traditional cell assays, the teams at Molecular Devices (CA, USA) and StemoniX (MN, USA) convened a research partnership that allows scientists to visualize and record neural activity in vitro, in small clusters of neural cells and then, due to the plug-and-play nature of the workflow, capture multiparametric characteristics of human neural cell function in ways and over timeframes not previously possible.
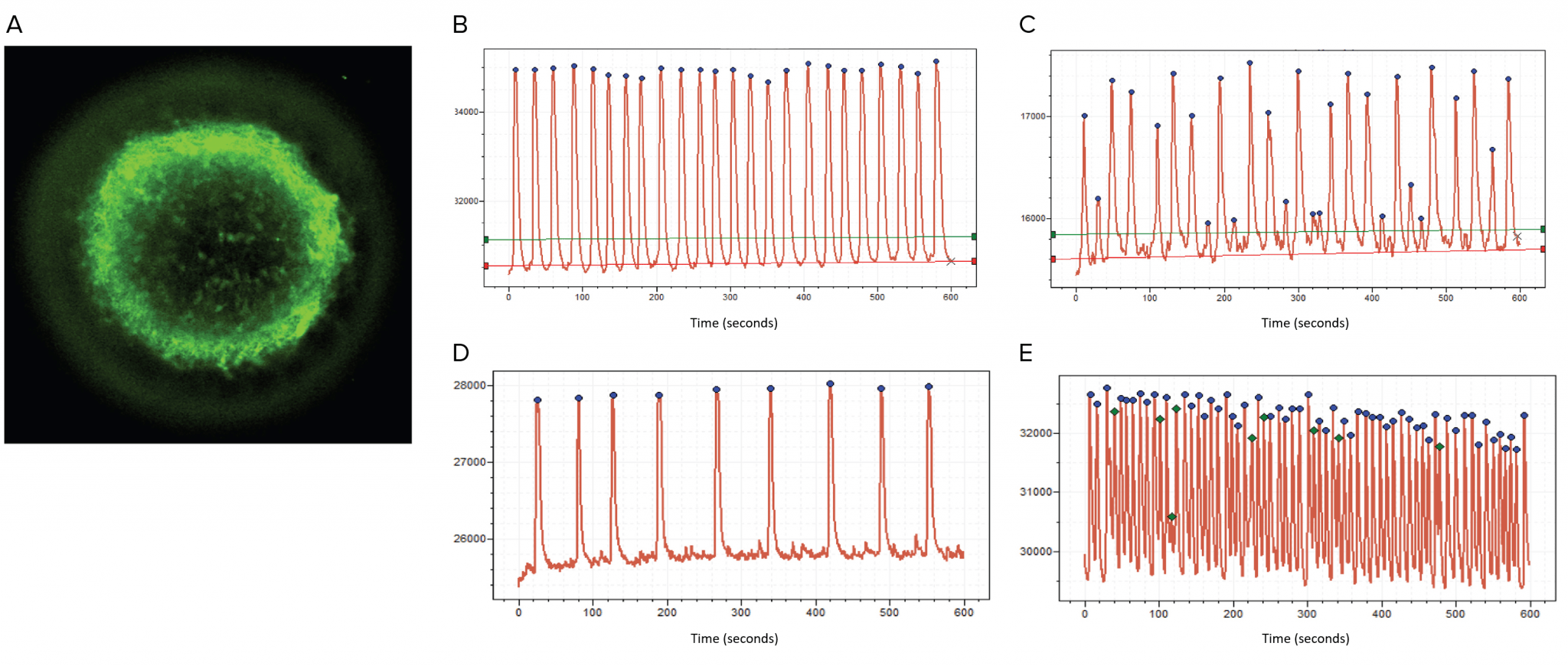
Figure 2. Neural activity can be imaged and interrogated as calcium oscillations. Still image of a microBrain 3D neurospheroid incubated with calcium dye (A). Spontaneous synchronous calcium oscillations recorded by FLIPR® Penta High-Throughput Cellular Screening System before (B) and after compound treatment (C, 3mM MK802). Peaks identified by software as blue dots. Oscillation patterns changed in response to neuromodulators (D, GABA; E 4-aminopyridine).
Our research leveraged microBrain® 3D, a human iPSC-based neural organoid platform, composed of mature cortical neurons and astrocytes in three-dimensional (3D) structures. The neurospheroids are functionally active and provided in high-throughput assay plates (Figure 1) [10]. Neural function is present in microBrain 3D spheroids as robust, spontaneous, synchronized, electrical and synaptic activity that is readily detectable as calcium oscillations (Figure 2). Using the FLIPR® Penta High-Throughput Cellular Screening System (Molecular Devices), calcium oscillations can be simultaneously recorded from all 384 wells. The waveform shapes dramatically change in response to neuromodulators (e.g., epinephrine, gabapentin, 4-amynopyridine) or in response to compounds with neurotoxic effects (Figure 2), and individual peaks and waveform characteristics and patterns can be quantified as numbers and concentration curves. Further, neurospheroids can be created from iPSCs obtained from either healthy “control” donors or from those with known neurological diseases. Differences in the Ca2+ oscillation waveform shape, or phenotype, of the patient-derived neurospheroids can be used as a biomarker for the disorder and to identify potential therapeutic compounds that ‘rescue’ the disease phenotype [12].
The system can detect functional, therapeutic or neurotoxic effects of various substances and can be used as a rapid assay to test multiple compounds. Using advanced fast kinetic fluorescence imaging and software analysis methods, differences between patterns under various conditions are easily identified and quantified by using more than 20 descriptors, thus allowing a direct and rapid comparison of potential compound efficacy or toxic side effects. In addition to the functional kinetic analysis described above, analyzing microBrain 3D with static automated 3D imaging technology allows our teams to ‘see’ through bundles of cells to get accurate morphological and structural data.
To characterize the system, our research teams used a set of neuromodulators with known mechanisms of action. The assay allowed detection of even small deviations from the control pattern of oscillations in a way that corresponded to expected mechanism of action. For example, GABA slowed oscillation frequency, while the potassium channel blocker 4-aminopyridine increased oscillation frequency (Figure 2). Potential compound-induced neurotoxicity was then explored by examining the neuroactive profile of more than 100 compounds. These included pharmaceutical drugs with known neurological effects and a panel of various environmental agents, such as pesticides, metals, flame retardants and other chemicals [10]. Importantly, analysis allowed the team to characterize peak patterns and provide a table of various descriptors including peak frequency and amplitude, characterization of irregularity, secondary peaks, gaps between peaks, and rise and decay times. After exposing the neurospheroids to each of the neuromodulators and neurotoxins, we determined effective concentrations of various compounds for causing deviations from the control untreated neurospheroids and ranked the compounds according to their effective potencies based on in vitro activity. Such ranking of multiple compounds allows researchers to flag compounds with potential neurotoxicity and prioritize those for more targeted investigations.
An example of how the system can be used to identify new approaches for treating neurological disease is shown by a focused study on Rett syndrome recently conducted by StemoniX. Healthy (control) and patient-derived neurospheroids were exposed to different compounds, and the impact and ability to rescue the disease phenotype was assessed using Ca2+ fluorophores and advanced fast kinetic fluorescence imaging and software. The phenotypic screening identified multiple target pathways, revealing – quickly and early in the process – what compounds signaled promising potential treatments for this neurodevelopmental disease. In practice, this approach may suggest more effective treatments earlier in drug development for increased success in the late-stage clinical trial phase and demonstrates that we are on the cusp of direct analysis of human-specific cellular mechanisms and subsequent patient-specific drug discovery [11].
Combining relevant human biology, high-throughput screening methodology and advanced analysis in this way has enabled quick, reliable detection of neurotoxic hazards and potential therapeutic pathways, giving researchers insight into how certain compounds found in both the environment and novel drug candidates might lead to devastating neurological disorders, as well as to potential cures.
The future of neurobiology research
Stated simply, a human iPSC-derived 3D neural culture assay complemented with state-of-the-art screening and imaging solutions offers researchers a promising biologically relevant platform for toxicity screening, disease modeling and drug development. This joint approach empowers neuroscientists to perform relevant high-throughput screening of drug candidates prior to more expensive, low-throughput animal and clinical trials – enabling compound stratification that weeds out potential toxic compounds and identifies the most promising therapeutic structures early in the process. Ultimately, this technology could enable physicians to prescribe more effective and personalized interventions that may vastly improve patient outcomes.
Learn more about how Molecular Devices and StemoniX are partnering to help advance discovery in neurology here.
Author information
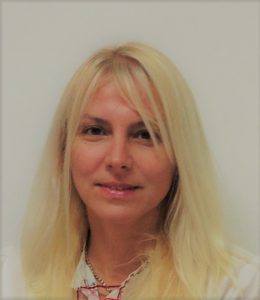
Oksana Sirenko
Oksana Sirenko, Ph.D., is a Senior Scientist at Molecular Devices specializing in complex cell-based model development for research and compound screening. With over 10 years of industry experience, she explores the use of 3D cell models – including organoids and organ-on-a-chip technologies – for cancer, neurotoxicity, angiogenesis and other complex biological process studies. Sirenko has authored 30 scientific papers.
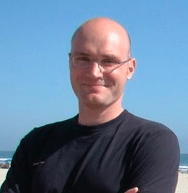
Cassiano Carromeu
Cassiano Carromeu is the Director of Research and Development at StemoniX and is an expert in neuroscience and stem cell biology. His post-doctoral training at UC San Diego (CA, USA) modeling autism in vitro helped to unveil some key aspects of Rett Syndrome and MeCP2 Duplication Syndrome. He joined StemoniX in 2016, where he has translated his expertise into products with the goal of facilitating drug discovery in neuroscience. His efforts led to the current microBrain products offered by StemoniX.