Would spider-man make a good stem cell biologist?
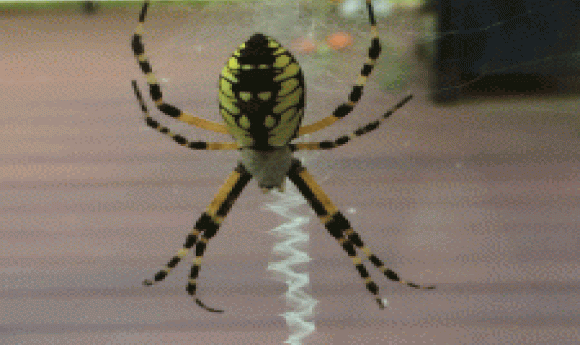
Spider silk, with its unique properties, is not only useful for superheroes, but it may actually turn out to be the key to regenerative medicine.
Writing Spider (Argiope aurantia)
Photo courtesy of Delphine Dean, Clemson University
Stem cells hold a lot of potential for regenerative medicine. Each year, scientists discover how to better model complex diseases in the laboratory using these cells. Why is it then that scientists still have a long way to go before they can translate their discoveries into clinical regenerative medicine?
When researchers use stem cells in the lab to model tissues and diseases, there is a limit to how much they can recapitulate. Tissues in living organisms are dynamic, three-dimensional systems, while research in the lab is often limited to flat, plastic dishes. Disease-in-a-dish models need more extracellular matrices and scaffolds to better mimic tissues in the body. In addition, many of the reagents scientists use are animal-based, which is immunologically unfavorable for medical treatments.
Spider silk is already helping biomedical sciences around the world. Not only is it strong and expandable, it is biocompatible with biomedical projects. In fact, in 2011, researchers from Hannover Medical School used spider silk to guide nerve regeneration in sheep. Ten months after the initial nerve graft, the scientists observed that the silk improved Schwann cell migration as well as axon regeneration and myelination (1).
The key component of spider silk is a protein called spidrion (2). Once isolated, researchers can use it to develop 2D film and 3D foam and mesh structures for cell culture systems, including those needed for growing stem cells and for tissue engineering. Scientists can grow stem cells on extracellular matrices such as laminin or vitronectin, and can develop 3D cultures, but the challenge is developing a system that enables 3D culture and is xeno-free, which are both necessary for developing translational medicine.
“I think spider silk is the most fascinating material there is. I mean, not only in the material itself, but how spiders manage to produce this material,” said Anna Rising, a professor at Karolinska Institute and the Swedish University of Agricultural Sciences. “Spiders can actually produce quite a lot of these sticky and aggregation-prone proteins. They can keep them in solution at extreme concentrations in the glands without having precipitation, and then they spin this fiber that is the toughest fiber that is known to man within just seconds or fractions of a second within their spinning apparatus.”
Working with spider silk, as powerful as it may be, is also a bit tricky. While Spider-Man may have a large supply of this silk at his fingertips, this is unfortunately not the case for scientists.
Acquiring Spider Silk
“So the hard part with spider silk, and why we don’t just use it in a lot of biomaterials and make clothing out if it or whatever, is unlike silkworm silk, it is really hard to mass produce,” explained Delphine Dean, an associate professor at Clemson University. “You can’t just have a bunch of these spiders next to each other. They will eat each other!”
Some research is conducted on native spider silk, but scientists can only extract a limited amount. Many scientists instead resort to recombinant synthetic spider silk fibers expressed in an array of organisms, from bacteria and yeast to plants or mammalian cells (2). Unfortunately, even this method is not that simple.
The spidrion sequence is highly repetitive—including up to 100x tandem repeats—and flanked by nonrepetitive N- and C-termini (2). This does not bode well for researchers trying to make long fibers. Natural and synthetic spider silk easily self-assembles and can therefore become insoluble. Designing, cloning, and expressing these sequences can be messy and lead to detrimental errors in the fibers (2). That, in turn, can make experimental reproducibility difficult; variability in biological reagents adds an unwelcome level of complexity.
Working to resolve these problems, some scientists modify the silk fibers. For instance, in 2007, Rising and My Hedhammer from the KTH Royal Institute of Technology identified a minimal spidrion motif called 4RepCT (2). This miniature spidrion is made up of just four tandem repeats and the nonrepetitive C-terminal domain. To maintain solubility, scientists fused the miniature spidrion to another protein, thioredoxin. When released, 4RepCT can spontaneously form silk-like fibers.
More recently in 2017, Rising’s team presented an improved synthetic spider silk along with a spinning process for more efficiently producing protein similar to the native silk (4). They created a chimeric recombinant silk protein, NT2RepCT, that included the soluble N-terminus from one spider and the soluble C-terminus from another. This helped improve the overall solubility of the synthetic spindrion. The scientists then created a silk spinning system in which NT2RepCT traveled through a thin pulled-glass capillary into an acidic bath, better mimicking the process of native silk formation. They incorporated the important shift in pH which was necessary for fiber formation. This process both improved the quality of the synthetic silk and also increased the amount of protein produced.
The more researchers understand spider silk biology, the closer they get to developing more efficient and biologically useful synthetic fibers. These fibers can be helpful in developing cell culture systems for modeling, drug testing, and ultimately, in regards to stem cells, applicable to regenerative medicine. For instance, the silk can be used to develop scaffolds for cells to grow upon. However, before scientists apply the silk to medicine, there are a lot of fundamental questions left to be answered.
“We don’t even know why these cells grow on the silk,” noted Rising. “We only know that they do. We should probably figure out how they attach to this material and how it affects the cells.”
Hoping to answer similar questions, Dean and her team compared how dental pulp stem cells grew on native and synthetic silk and evaluated how they affected the cells (3). Cell viability and fluorescent staining of the cytoskeletons did not show any differences. However, her team noticed better cell adhesion and alignment on native silk. Imaging the fibers showed that the natural silk they isolated was consistent in fiber diameter, while synthetic fibers were larger and exhibited variability in size. Interestingly, cells grown on synthetic silk hydrogels exhibited better cell viability, attachment, and growth than those grown on synthetic fibers alone, which came as a surprise since the content of the synthetic fibers and silk hydrogels are similar.
“Some of the properties of how the cells interact with the proteins are maintained, but some of it we lose when we try to make these fibers,” said Dean. “You can just tell from the geometry. We are just not as good at making fibers as the spider is right? Spider silk fibers are very small in diameter and we haven’t gotten quite to that point when it comes to the manufacturing side.”
Scientists like Hedhammar try to engineer silk with additional proteins in attempt to both better understand its basic biology and ultimately to improve the system for cell culture and tissue engineering. She explained that the dimensions and mechanics of the fibrils are important and need to match real tissues.
“When cells can really attach and make these focal adhesions, these gathering of several receptors to something that, to the cell, looks like the extracellular matrix, that is when the cells get the signal, ‘hey I am in the right place to be a tissue,’” she said.
Spiders and Stem Cells
In 2006, David Kaplan and his team at Tufts University modified spider silk and improved stem cell culture. His team cultured human bone marrow-derived mesenchymal stem cells on spider silk fibers modified to include a cell binding sequence. After several weeks, the team noted an increase in calcium deposits, an indication of bone differentiation, proving that the silk could enhanced differentiation in this system (5). 6 years later, Rising used the 4RepCT in both 2D (silk film) and 3D (silk foam) systems to develop a defined culture system for rat neural stem cells. Cells cultured on these fibers remained undifferentiated and viable. However, when initiated, the cells could differentiate into multiple neural cell fates (6).
The Hannover study demonstrating sheep nerve regeneration and the studies with mesenchymal stem cells prove that spider silk may be a key in regenerative medicine. Working towards the goal of creating a xeno-free culture system, Rising’s team developed a xeno-free stem cell culture system, a requirement for translating these studies to human clinics.
Since other extracellular matrices such as laminin, fibronectin, and vitronectin have proteins favorable for stem cell culture, Rising’s team developed modified 4RepCT proteins fused to proteins from other matrices. This assay highlighted a version of 4RepCT fused to a protein from vitronectin. Over long-term culturing, stem cells maintained pluripotency, could be passed efficiently, and still retained the potential to differentiate into all three germ layers (7, 8).
Spider silk holds a lot of potential for stem cell research, regenerative medicine, and biomedical sciences as a whole. However, the field is still very young and there is much more that needs to be done. To reach into translational medicine, the technology needs to grow.
“I hope we can scale up the production. I hope we can make fibers that are as strong as native silk, and I hope we can control the fiber production process in a way that we can make very defined three-dimensional matrix for stem culture,” Rising said. “I think the 3-dimensional environment is much more important for the cells than previously acknowledged.”