Fighting cancer with deadly bacteria
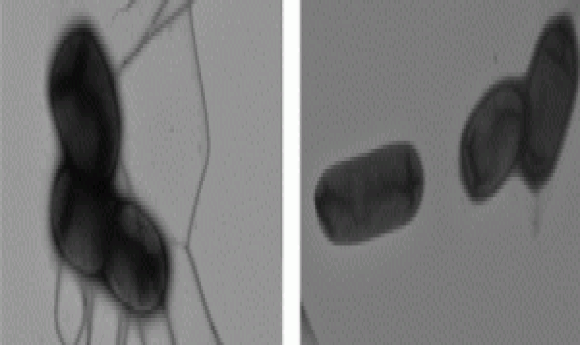
A 200-year-old observation might provide a new way to eliminate tumors. But it also means infecting cancer patients with Salmonella.
Electron microscopy of Felgner’s team’s wild type and mutant Salmonella strains showing decreased flagella in the mutant rendering them less motile (3).
Diagram of hypoxia inducible expression of p53 and Azurin designed by Bellamkonda’s group (4).
Two hundred years ago, when physicians routinely traveled with leeches, glass cups, and candles for bloodletting, they noticed something peculiar—bacterial infections sometimes preceded remission or even complete elimination of cancer. While many ideas from this era have been dismissed as unreliable, the alluring question of whether or not bacteria affect tumors has proven irresistible to some researchers, and their curiosity is beginning to pay off.
American physician William Coley was the first to experiment with bacterial treatments for cancer in the late 1800s. By injecting his patients with various combinations of live and heat-killed bacteria, he eventually landed on a mixture he called “Coley’s toxin” that caused regression of a majority of tumors, sometimes even entirely eliminating them. This treatment remained in clinical use for sarcomas until 1963.
Since then, scientists have identified several bacterial species that naturally home in on tumors, grow and replicate inside them, and eventually kill the cancer cells. However, “Bacteria like Salmonella are pathogens,” noted Sebastian Felgner from the Helmholtz Center for Infection Research in Braunschweig, Germany. “Thus, designing a bacterial vector strain for cancer therapy based on Salmonella requires an appropriate balance between safety and therapeutic efficacy.”
With genome-editing technologies now readily available, Felgner and other researchers are creating new strains of live bacteria, inching ever closer to the balance required for bacterial combatants—on their own, teamed with the immune system, or carrying anti-cancer payloads—to eliminate cancer in humans.
Ties to Immunity
Early tests showed that some strains of Salmonella preferentially colonized solid tumors and readily killed cancer cells, with Salmonella typhimurium showing special promise. It’s not safe to inject this pathogen into already immunocompromised cancer patients, so researchers at Yale University took aim at its virulence and septic shock potential by deleting portions of key genes (1).
The resulting S. typhimurium strain maintained the ability to target and kill tumors but stayed in the blood for only 24 hours in monkeys, making it much safer than the original. Animal testing showed so much potential that this bacterium progressed rapidly into Phase I clinical trials in late-stage melanoma patients (2). Unfortunately, “[The bacterium] was not able to colonize most of the human tumors during the clinical trials in 2002 as the strain was over-attenuated for the human immune system,” Felgner recalled.
Felgner attributes these disappointing results in part to pre-existing immunity. “Most laboratory animals are kept under specific pathogen-free conditions. In general, they have not encountered the bacterial vector before and thus do not exhibit any immunity against it,” he explained. “On the contrary, humans might have experienced the bacterial vector before and therefore might possess immunity against the bacterial agent.” Such immunity would enable humans to clear the bacteria quickly, possibly before damage is done to the tumor.
While it’s not clear exactly how the bacteria kill tumor cells, researchers suspect that recruiting immune cells to the site contributes significantly. The patients in the study had previously received chemotherapy, leaving them immunocompromised, which also may have contributed to the poor response.
Back to the Beginning
On the heels of the 2002 clinical trial, researchers returned to the bacteria. They needed to keep the bacteria safe for the patients’ normal tissues but not safe for the tumor cells, which needed to be aggressively attacked. “In the aftermath, it turned out that this strain carried a large gene deletion that rendered the strain amotile, thereby losing an important stimulant for the innate immune system,” Felgner explained.
Armed with this information, his team decided to attenuate the bacteria differently by focusing on lipopolysaccharide (LPS) synthesis. LPS is essential for bacterial survival, but it also causes sepsis in humans. By targeting genes involved in LPS synthesis, the team shortened the LPS structure, rendering the bacteria safe for therapy but also unable to combat tumor cells.
“The bacteria were too weak,” Felgner said. “For that reason, we thought it might be beneficial to generate a conditionally attenuated system where the bacteria become attenuated in vivo over time.”
To accomplish this, the team used arabinose-inducible promoters to control wild-type LPS genes. Without arabinose, LPS disappeared within 3 hours. They grew their modified Salmonella in the presence of arabinose to express wild-type LPS and injected this strain into tumor-bearing mice. Although mouse health suffered more with these bacteria, the mice survived the treatment and showed tumor cell death (3).
Meanwhile, Ravi Bellamkonda and his colleagues at Duke University focused on restoring motility in the strain of Salmonella used for the 2002 clinical trial. To accomplish this, they mutated a gene essential for purine biosynthesis, meaning the bacteria would require an outside source for purines. As it happens, tumors are a rich source of purines, while healthy tissues have undetectable purine levels.
To these tumor-seeking bacteria, they also added the ability to express the tumor suppressor protein p53 along with the protein Azurin, which stabilizes p53 and induces apoptosis in tumor cells. The loss of p53 function associates with more than 50% of cancers with poor clinical prognosis, motivating researchers to restore its function as a form of cancer therapy. The researchers placed these genes under the control of a tumor-specific promoter that was previously identified by Felgner’s group; now, the proteins would only be made in the low-oxygen environment within a tumor.
Bellamkonda’s team administered their bacteria to a group of six rats with glioblastoma (GBM), an aggressive cancer where the goal of treatment is to extend life; recovery is considered out of reach (4). When Bellamkonda looked at the response, he felt “optimistic, but also skeptical,” he said. “You could imagine, seeing only one rat respond in each group as hopeful, but not necessarily robust. So we repeated several more times, and by the end of our study, we had treated 36 rats, 7 of which had evidence of complete tumor regression. When I saw the consolidated data, I was greatly pleased.”
“Achieving overall survival means that—even with this being our first shot at a bacterial therapy for GBM—we’ve struck upon the basis for something very much needed in the clinic: a drug delivery system to the brain that can reach previously undeliverable regions,” he added.
In the rats that didn’t respond to treatment, Bellamkonda and his colleagues saw that portions of the tumors were colonized and cells in those regions had died through p53-directed apoptosis. The team hypothesized that after colonization the tumor continued growing rapidly, allowing newer cells to escape the therapy.
“This approach could be very promising,” Felgner said. “However of note, [the bacteria used in the clinical trial] was not able to colonize most of the human tumors during the clinical trials in 2002 since the strain was over-attenuated for the human immune system. Thus, other strains need to be investigated to possibly achieve better results in humans.”
Pressing Forward
According to Bellamkonda, drug studies showing significant survival in mice and rats required daily injections where the drug travels throughout the body, meaning that treatment comes with significant and devastating side effects. “With bacteria, the delivery can be as infrequent as once,” he said. “There is still work to be done refining the bacteria so that this is 100% reliable, but that is the path this technology is taking us.”
Researchers currently understand much about how bacteria travel to the tumors and trigger innate immune responses once they arrive. But details of the bacteria-induced anti-tumor response remain unknown. Felgner said filling in this knowledge gap is essential for accomplishing complete tumor and metastasis clearance. “We are absolutely convinced, though, that this can eventually be achieved,” he said.
Obtaining the genome sequences of all experimental bacteria should propel future work, and conducting tests in mice that carry functional human immune systems could help predict how any newly developed bacteria will perform in humans. “Nevertheless, an absolute prediction of translation from animal to human might be still difficult,” Felgner said.
Bellamkonda is exploring other pathways for inducing apoptosis in the hunt for robust anti-tumor responses and is working out dosing schedules to balance side effects. His team plans to test their Salmonella strain in other tumor models. They are also interested in developing ways to promote bacterial exploration of the tumor environment after colonization to keep bacterial expansion up to pace with tumor expansion.
“There are so many brilliant people working in the microbiology area today, and there are a lot of promising ways to start using these [bacteria] to treat cancers in ways we couldn’t have imagined 10–20 years ago,” Bellamkonda said. “We opted for Salmonella, but there is a plethora of different bacterial strains available, each with its own particular features, just waiting for the right application.”