Ask the Experts: glia characterization
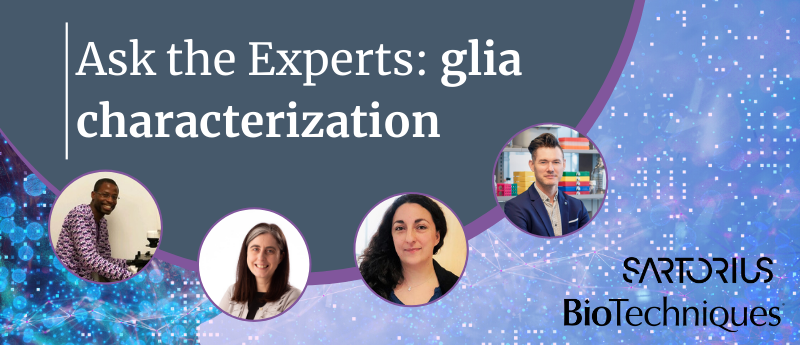
Glial cells, such as microglia, astrocytes and oligodendrocytes, play a number of key roles in the central nervous system (CNS) but are often overlooked. However, the field of glial biology is expanding, and in recent years there have been major advancements and novel tools have been developed to further glia research.
In this ‘Ask the Experts’ feature, Susana Alcantara (Sartorius; Göttingen, Germany), Shane Liddelow (New York University; USA), Ukpong Eyo (University of Virginia; USA) and Nicola Allen (Salk Institute; CA, USA), discuss their perspectives on key advances, current obstacles and future developments in the field of glial biology.
Download this feature as a PDF
Glia questions:
- How has our understanding of glial cells and their role in health and disease developed over the past decade?
- What are the challenges faced when investigating glial cells?
- Why is it important to characterize glia?
- What methods are available for characterizing glia?
- What insights into the CNS can the characterization of glia give us?
- How can this knowledge be translated into the clinic?
- Can, or could, glia characterization be used for diagnostics?
- What are your predications for the future of this field?
How has our understanding of glial cells and their role in health and disease developed over the past decade?
Susana Alcantara (SA): Neuroglia as a term first appeared in the 1850s, following previous independent identification of several glial types. The field developed, and it wasn’t until 1919 when P. Del Rio-Hortega described the term glia as we currently understand it. Nevertheless, we had to wait until the 1980s for glia related publications to really take off, in parallel to novel Molecular Biology and the development of calcium imaging techniques. If we focus our attention on the last 10 years of research, one can see that the neuronal-glia crosstalk was highlighted, and glial function shifted from a mere supportive to an active role in processes as diverse as neurogenesis, synapsis homeostasis, neuronal connectivity, surveillance, blood brain barrier permeability or myelination, among others. Whilst not novel, the link between neuroglia and brain homeostasis was strengthened, enabling a more detailed perspective on the role of glia in disease.
Shane Liddelow (SL): In so many ways! We now have a thorough understanding of how synapses are formed, matured and activated – largely due to investigations of astrocyte biology. We are coming to terms with how microglia and other peripheral immune cells interact with astrocytes to drive degeneration of neurons and respond to pathology in a wide range of diseases like Alzheimer’s and Parkinson’s disease, and demyelinating diseases like multiple sclerosis. We also understand the transcription factors and protein machinery required for proper wrapping and compaction of myelin sheathes. And, if we broaden our scope to include blood vessels and pericytes, we have learned about region-specific control of blood flow and nutrient transfer throughout the central nervous system.
Nicola Allen (NA): In addition to glia being the cells that express many of the risk genes for neurological disorders, the recent increase in transcriptomic analysis of different cell types in the brain has made it clear that in many neurological disorders, particularly those associated with age and neurodegeneration, the most profound alterations in gene expression are occurring in glial cells. In particular, at early disease stage it is microglia and astrocytes that are altering their gene expression, rather than neurons, suggesting that glia should be the target of therapies designed to slow disease progression.
Ukpong Eyo (UE): Our understanding has grown markedly. New tools have been developed that have allowed us to better interrogate the roles of these cells. For example, in my subfield of glia involving the study of microglia, transcriptomic analysis has provided a more comprehensive view of these cells and how they change their states in various conditions. More specific conditional approaches have been developed to selectively target these cells genetically as well as label them. Pharmacological tools have been employed to interrogate whether these cells play roles in various health and disease conditions. Imaging modalities have been developed to visualize their activity and changes in development, maturity and disease. Glia are no longer an “after thought” in neuroscience research as they seemed to be at the beginning of this century. They are strongly considered in CNS physiology and pathology.
What are the challenges faced when investigating glial cells?
SA: Neuroglia includes cells from both the central (astrocytes, microglia, oligodendrocytes and epidermal cells) and the peripheral (Schwann and satellite cells) nervous systems. These cells are very diverse both morphologically and functionally. In addition, there is a temporal nature linked to their phenotypes. Whilst very informative, this dynamism is lost when exclusively using end-point analysis. Studying glial cells in an in vitro setting presents additional challenges linked to the sensitivity and reactivity of these cells, causing either a deleterious or an activated state different to that within the healthy brain.
Incorporating live-cell analyses into a research workflow has the potential to monitor and measure dynamic cell behaviors and phenotypes in a stable environment with minimal perturbance and over long periods of time. This allows data-driven decisions to be incorporated within the routine experimental workflow ahead of potential downstream end-point analysis.
SL: Like many fields, the study of glia has been somewhat hamstrung by a lack of tools that are sufficiently specific to address the exciting questions we are all asking. There have been amazing advancements in the past decade however – the development of serum-free culture systems, new genetic driver lines, and the explosion of tools and models in the invertebrate animal world, not to mention stem-cell based technologies. All of these tools, combined with an exciting array of datasets, have helped to overcome these challenges.
Moving forward, the main challenge that exists is making sure this amazing array of tools are accessible and continue to be taken up by the field.
NA: A major challenge with studying glial cells is a lack of tools designed to specifically manipulate them, rather than repurposing tools designed for use in neurons. This is beginning to change, for example with more physiological methods to manipulate intracellular calcium, and is an exciting area of innovation.
UE: One of the challenges the glial field is facing is appropriately defining glia. Traditional approaches have used specific proteins to identify different glial subtypes. However, once thought to be specific and/or comprehensive, some of these markers are now known to be more promiscuous for other cell types or not comprehensive to define glia in development or disease. Moreover, with the increasing appreciation of glial heterogeneity or diversity, great care has to be undertaken by the field in appropriately defining glia. There is progress being made here. Earlier this year, a consensus paper was published by leaders in the field on defining astrocytes and one is ongoing on defining microglia. Presumably, this will filter into other glia. Finally, training the field to “unlearn” previous (inadequate) nomenclature and definitions is not going to be a trivial task given the replete glial literature of especially the 21st century. This is a philosophical challenge that is being informed by experimental data.
A second challenge that flows from this first challenge is, considering the difficulty of accurate molecular definitions, adequate molecular approaches to target glia is difficult. For example, at present, oligodendrocyte precursor cells are a glia that is generally identified using at least two markers that are independently expressed by other cells. This makes it difficult to generate mice with specific genetic oligodendrocyte precursor cell identity and so slows down progress.
A third challenge with investigating glial cells is that they have a high capacity to change “states”, especially when removed from the living animal. This often makes it difficult to study them since they are best studied in their native environment in vivo. Moreover, with their capacity for change, they are not always the same in various disease conditions, making it difficult to extrapolate findings from one disease into another. Therefore, characterizing glia requires great experimental care and precision.
A fourth challenge to studying glia is that the wealth of our knowledge of glia is derived from animal (especially rodent) models which may not always recapitulate the human phenotype. Finding appropriate ways to study human glia in their native CNS environment is of course difficult.
Why is it important to characterize glia?
SA: The ratio of glia to neurons in the brain is around 50 to 1. The diversity and dynamism of neuroglia makes them imperative in crucial roles, such as developing nervous systems, maintaining brain homeostasis or regulating energy metabolism. Under pathological conditions, such as injury, neurodegeneration or infection, the different glia play a key role in regulation and repair, delaying disease progression. Characterizing glia will help the path to understanding brain development, disease and injury, and essentially it will need to be included in appropriate therapeutic and treatment strategies.
SL: It is becoming apparent that glia are very early indicators and drivers of a whole range of neurodevelopmental, neuropsychiatric and neurodegenerative diseases. This is in addition to being integral contributors to normal brain development and function. As scientists, if we are to fully understand brain function during health and disease, and if we hope to develop novel therapeutics for currently untreatable diseases, it is apparent that we must study and understand glial function.
NA: In mammals, glial cells collectively make up 50% of the cells in the brain. Therefore, as with any tissue in the body, understanding how the brain functions in health and disease requires analyzing all of the component cell types together, rather than just focusing on the output cells i.e. the neurons.
UE: In the wider public, glia are not as well-known but it is important to understand and characterize them because they make up half (and sometimes more than half) of the cells in the CNS. We cannot properly understand the CNS without adequately, accurately and sufficiently characterizing a central portion of the cells that make it up. Moreover, since glia are often dysfunctional in disease, it is likely that better understanding glia will revolutionize our abilities to treat neurological disorders and dysfunction.
What methods are available for characterizing glia?
SA: A large plethora of in vivo and in vitro research methods to characterize glia are currently in use. From the cell-based in vitro perspective, methods based in optimizing isolation and culture practices to minimize glial perturbance and activation, particularly important for microglia, are currently in focus. Regarding glia characterization, several methods are available, from the perspective of morphological diversity, health and proliferation, migration or neuronal modulation. These methods consist of a combination of genomic (sequencing, genetic modifications), proteomic (western blot, flow cytometry, immunocytochemistry, ELISA, mass spectrometry) and functional identification (electrophysiology, imaging techniques, calcium sensors, cytotoxicity, optogenetic modulation), just to name a few. All these methods have in common that they are endpoint and cell exhausting. As a result, a large number of resources are needed to characterize a kinetic process. This is where non-invasive real-time live-cell analysis is redefining the possibilities and workflows of cell biology. A series of time-lapse images acquired and automatically segmented using systems such as the Incucyte® Live-Cell Analysis System can answer some of these research questions in an upstream and non-perturbing manner, ahead of any necessary end-point analysis, incorporating the kinetic nature of biological processes to multiparametric analyses.
UE: There are various methods that are available to characterize glia. Some are traditional approaches and others are more recent developments. One example of a traditional approach is the use of molecular immunohistochemistry to characterize glia. Others include immunological flow cytometric techniques as well as biochemical methods. An example of a more recent approach is the use of RNA sequencing technologies to understand glial states. Furthermore, new genetic fate mapping and conditional molecular knockout or overexpression have also been employed. In addition, over the last decade, researchers have employed the use of chimeric mice to further characterize human glia in a live rodent rather than in vitro setting. These are all very useful methods to characterize glia.
What insights into the CNS can the characterization of glia give us?
SA: An understanding of glia in relation to health and disease of our nervous system will help elucidating the intricate balance within our CNS and thus recovering homeostasis following disease and injury. I particularly find it interesting the insights into neuron-glia communication within the tripartite synapses, how glia modulates signal transmission across neuronal networks and the role of non-overlapping regional populations of astrocytes.
SL: This one is easy! An understanding of glia enables us to understand the intricacies of how the central nervous system is built, how it functions and how it breaks down. An understanding of glial contributions to these stages enables us to better diagnose and treat disease, and where therapies do not exist, to make strides towards producing novel therapeutics.
UE: As components of the CNS, understanding its function would require us to understand and characterize glia. Although neurons are the “principal” electrically active cells of the CNS, we now know that glial function is critical to neuronal function. For example, one group of glia, the oligodendrocytes, produce myelin that facilitate the conductance of neuronal impulses. These allow vertebrate with myelin to think, move and react fast. Another group of glia, the astrocytes, help to regulate the number of neurotransmitters that are available in the extracellular space in the brain. This language needs to be finely tuned: too much is bad; too little is bad. One can imagine that in diseases or pathologies where there is poor neuronal communication, it could potentially be improved by enhancing glial functions. It’s an intriguing idea with exciting prospects.
How can this knowledge be translated to the clinic?
SA: Translation from an in vitro setting to the clinic is a process that certainly needs optimizing. Special challenges are inherent to the neuroscience field. I believe that a good step forward may come from combining advanced cell models with specialized analysis tools and improved computational technology. In our group, we focus on the development of kinetic cell models and automatic phenotypic quantification using live-cell analysis. We combine expert scientists, software and hardware engineers to simplify and optimize methods to objectively quantify meaningful biological processes.
UE: Many neurological diseases exhibit dysfunctional glia, for example multiple sclerosis, Alzheimer’s disease and glioma, indicating that characterizing and understanding glia could facilitate the development of novel and/or complementary treatment strategies for these and other diseases. As an example, it is now clear that microglia are seriously dysfunctional in Alzheimer’s disease and this is likely to be, at least in part, a cause (rather than merely a consequence) of the disease progression. Because microglia are also unique immune cells in the CNS, they have unique proteins that could be targeted without direct effects on neurons. These can be developed in the lab. Once this is shown to work at the bench, approaches to develop human-sensitive drugs can be employed for the clinic. There is much excitement that could be had from more research into glia and its subsequent translation to the clinic.
Can, or could, glia characterization be used for diagnostics?
SA: Scoping optimal non-invasive techniques within the field of diagnostics for CNS disorders, if interpreted as an ongoing evaluation of disease progression, is imperative. Current efforts in the areas of ischemic stroke, glioma therapy and neurodegenerative disease diagnosis successfully use plasma or cerebrospinal biomarkers. This field is very promising and focusing on glial biomarkers may prove invaluable for the future understanding of these diseases.
UE: This is a great question. Because glia are known to be altered in disease, this could be a useful approach. In fact, because glia are often sensors of deviations from normalcy as occurs in disease, glial-based diagnostics could be promising to early detection of disease pathology. But this approach seems to be at its infancy, though it will hold a lot of prospect for the future.
What are the predictions for the future of this field?
SA: Overall, I believe that the development of advanced cellular models, such as iPSCs derived from glial cells, studied in the context of complex cultures, from 2D monocultures to polycultures, and from 3D spheroids to brain organoids, will improve translation. When those models are coupled to advanced technologies with appropriate throughput, and optimized data handling, the field of neuroglia basic research, as well as related applied developments, will see a great advance. Whilst it is early days for glia cell-based therapies, several potential targets have been successfully identified, for example, Riluzole for Alzheimer’s disease and amyotrophic lateral sclerosis, Fingolimod for multiple sclerosis, or hIL13-PE for glioma. In more general terms, modulation of the blood–brain barrier is very appealing for the drug development market. The field of glial cells as therapeutic targets comes with high expectations for novel developments. In silico modelling, as well as adapted software tools, will also have an impact in extracting the maximum biological data possible from these very dynamic cell types and disease models. I am excited to see how this field will develop and what improved therapeutics it may provide.
SL: As the implications for glia in the initiation and progression of disease continue to be uncovered, and more readily accessible tools and datasets come online, I see a continued resurgence in the number of researchers studying glia in health and disease. This will continue to be an exciting and paradigm shifting field, as we benefit from biologists, chemists, engineers and clinicians all working together for a common goal. The addition of immunologists and disease-specialists focusing their attention on cell-cell interactions in the brain that involve glia will continue to help unravel the complex web of mechanisms behind neurological and neurodegenerative diseases. What an exciting time to be working in glia!
NA: This is a great time to be a glial researcher. There is increased interest in these cells due to the strong link to neurological disorders, so, many new groups are entering the field. This will lead to an acceleration of discovery about the normal properties of these cells, as well as the development of new tools to allow their study, ultimately benefitting all glial researchers.
UE: It’s difficult to predict the future and only weather personnel are forgiven for wrongly predicting future events. That said, I think three predictions are safe to make. Glia will become (already are) commonplace in CNS research. I foresee that this will crystallize into a generation of (a) specialized glial focused journal(s) from the high impact Cell/Nature/Science group in the near future, perhaps the next decade. Second, there will be better clarification and consensus of glia nomenclature with the emergence of these consensus papers in the works. It is possible that formal glial committees and bodies will exist to discuss, moderate and define progress in the field. Finally, on the technical front, the research will develop to accommodate reliable in vitro 3D culture or organoid-type systems for the study of human microglia, in vivo chimeric mouse models for the study if glia in vivo and more specific approaches to target glial experimentally. These are exciting times to be involved in glial research.
Meet the experts
Susana L. Alcantara | Principal Scientist and Neuroscience Program leader within the Applications unit of BioAnalytics at Sartorius AG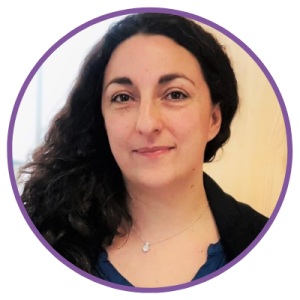
Susana L. Alcantara is currently a Principal Scientist and Neuroscience Program Leader within the Applications unit of BioAnalytics at Sartorius AG. She is a highly experienced cellular neuroscientist with over 15 years of experience in large pharma drug discovery (GSK; London, UK), biotech (Essen BioScience; MI, USA) and start-up (Proximagen; Cambridge, UK) R&D settings. Trained initially as a Biochemist & Microbiologist, Susana’s early work focused on translational research models and cellular signaling pathways in neurodegeneration and dyskinesia in Parkinson’s disease. Within the Sartorius neuroscience team, during the past 11 years, she has developed and validated a wide range of advanced cell assays for neuroscience to extend the suite of applications of live-cell analysis.
Shane Liddelow | Assistant Professor at New York University
Shane Liddelow is an Assistant Professor in the Department of Neuroscience and Physiology and the Department of Ophthalmology at New York University. He received his PhD with Katarzyna Dziegielewska and Norman Saunders in Pharmacology from the University of Melbourne (Australia). As a postdoctoral fellow in the lab of Ben Barres at Stanford University (CA, USA) his research focused on astrocytes, the major glial subtype in the brain. His most recent research showed that one form of reactive astrocyte is induced by factors released by microglia.
Shane was a recipient of the NHMRC (Australia) CJ Martin Training Award (2012-2016), the Glenn Foundation (AZ, USA) award for Aging in 2016, and was named a STATNews (MA, USA) Wunderkind in 2017. In 2019 the Alzheimer’s Association (IL, USA) awarded him the Inge Grundke-Iqbal Award for Alzheimer’s Research for the most impactful study published in Alzheimer’s research during the previous two calendar years. In 2020 he was awarded the David Hague Early Career Investigator of the Year Award by Alzheimer’s Research UK (Cambridge, UK).
Nicola Allen | Associate Professor at the Salk Institute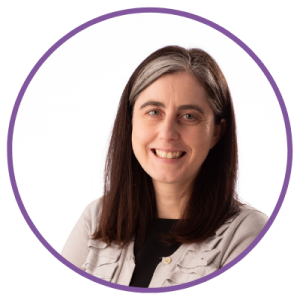
Nicola Allen is an Associate Professor at the Salk Institute for Biological Studies. She received her PhD working with David Attwell at University College London (UK) studying neuronal responses to ischemia and performed Postdoctoral research with Ben Barres at Stanford University (CA, USA) where she identified how astrocytes regulate the formation of functional synapses between neurons. Current work in her lab investigates how neuronal synapses are regulated throughout life: from the formation of synapses during development, to the remodeling of synapses in the adult in response to experience, to the loss of synapses in aging. This is approached not just by studying neurons, but by asking how non-neuronal glial cells, specifically astrocytes, regulate synapse number and synaptic function. The goal is to use this knowledge of astrocytes to repair synapses when they are dysfunctional in diverse neurological disorders.
Ukpong Eyo | Assistant Professor at the University of Virginia
Ukpong Eyo is an Assistant Professor at the University of Virginia. Eyo attended graduate school at the University of Iowa (USA) where he developed a keen interest in real-time imaging of microglia during development under the mentorship of Michael Dailey. During his time in the Dailey Lab, Eyo reported remarkable migratory capacities for neonatal microglia and elucidated purinergic mechanisms in microglial demise under simulated ischemic conditions. Following his PhD studies, Eyo joined the lab of Long-Jun Wu, first at Rutgers University (NJ, USA), then at the Mayo Clinic (MN, USA) to study microglial-neuronal communications. His postdoctoral research in the Wu Lab uncovered novel physical interaction phenomena between microglia and neurons. In August 2018, Eyo started his independent lab in the Department of Neuroscience and the Center for Brain Immunology and Glia at the University of Virginia to continue his research on microglia with a focus on the developing brain.
Disclaimers
The opinions expressed in this interview are those of the interviewees and do not necessarily reflect the views of BioTechniques or Future Science Group.
This feature was produced in association with Sartorius.