Finding a molecule in a haystack
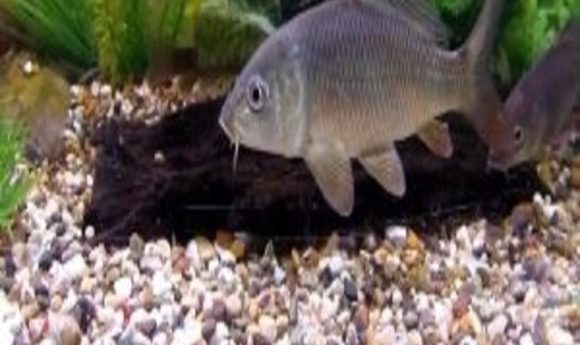
What do researchers do when they need to find very rare molecules in a sample?
When fishing derbies take place during the summer, competitors often wonder just how many fish are actually in the water and where they might be. While specific devices to locate fish are available and some professional anglers have learned to identify where certain species like to “hang out,” neither of these approaches can truly assess just how many fish are in a given lake at a particular moment. So what can be done? It turns out that there is a method for figuring out how much fish diversity exists in an aquatic environment. And the surprising part is that it involves using that PCR machine sitting in your lab.
The technique is called environmental DNA (eDNA) analysis, and the concept is fairly straightforward: quantitative real-time PCR (qPCR) amplifies the eDNA for a target species from a water sample, after which that data can be used to estimate biomass and abundance. For those at the fishing derby, researchers could sample the water, amplify the eDNA for catfish or bass, and then know just how likely a contestant was to win the derby with that prized bass, and where to float the boat for the best chance.
Obviously there are other, more important environmental uses for eDNA analysis when it comes to assessing biodiversity, so optimizing the technique is important. Traditional qPCR approaches have a downside since qPCR calculate a cycle threshold metric, which is an indirect measurement of DNA concentration. In 2015, Hideyuki Doi and colleagues from the Institute for Sustainable Science and Development at Hiroshima University in Japan decided to try moving beyond qPCR for eDNA analyses efforts to estimate fish abundance and biomass in environmental surveys by implementing a more quantitative way of measuring fish.
Droplets of eWater
For quantitative PCR analysis, droplet digital PCR is the way to go. In droplet digital PCR, DNA or RNA molecules are physically separated into individual reaction vesicles (one molecule per vesicle), or droplets, wherein each molecule is amplified and analyzed. Because every molecule is split up, the resulting amplification reactions not only identify the presence of a specific sequence in the sample, but can also provide a clear measure of the starting numbers of those target molecules – a true quantitative measure of target DNA in a given sample. Doi and colleagues realized that for eDNA analysis – droplet digital PCR might provide the path to better biodiversity assessment.
To find out, Doi’s team set up 12 different tanks of carp to act as aquatic mesocosms. The numbers of carp in each outdoor tank was varied, from a negative control tank with 0 fish to a tank with more than 85 individual carp. Before adding any of the carp to a tank, however, the researchers checked the water conditions and weighed each carp before and after the experiment to calculate the true biomass present in each of their experimental environments.
The results showed that both qPCR and ddPCR were able to quantify concentrations of carp eDNA in the different water tank samples. These techniques also assessed carp abundance and biomass in the tanks, with linear relationships over different numbers of fish. However, Doi’s group found a lower coefficient of variation when using ddPCR than qPCR to assess biomass and species abundance, especially at lower concentration ranges of eDNA. The authors concluded that when it comes to low abundance eDNA concentrations, ddPCR provides a more accurate approach for quantification and analysis.
Moving Beyond PCR
Clearly, qPCR and ddPCR are robust approaches for detecting and quantifying low abundance targets such as pathogens or rare genetic variants in samples. But these approaches involve amplification time and costs, which has led some groups to look beyond traditional PCR amplification approaches in the quest for sensitive pathogen detection and disease monitoring that is faster and less costly.
Broad Institute and Harvard University researchers James Collins and Feng Zhang along with their colleagues have been working on ways to advance the microbial CRISPR system for diagnostic applications. With its powerful ability to target specific DNA and RNA regions, and then cleave nearby nucleic acid sequences, CRISPR has been used in a growing number of molecular biology and now diagnostic applications.
Last month, Collin’s and Zheng’s team showed for the first time how a modified CRISPR system could detect nucleic acids with very high specificity and sensitivity. Their platform makes use of the Cas13a enzyme, which is an RNA-guided RNAse that can be programmed using CRISPR RNAs to target specific RNA sequences. Once a specific sequence is recognized, the Cas13a enzyme will cleave nearby, non-targeted RNAs.
To take advantage of this CRISPR/Cas13a system for nucleic acid detection, the researchers need a way to a create signal when the target sequence had been identified. For this, they added a quenched fluorescent reporter RNA to their reaction that would flouresce upon cleavage by Cas13a. With some additional modifications involving a single isothermal amplification step, the system, which the researchers called SHERLOCK (Specific High Sensitivity Enzymatic Reporter UnLOCKing), attained attomolar sensitivity and single base pair resolution – requirements that are necessary for many diagnostic applications.
“This tool offers the sensitivity that could detect an extremely small amount of cancer DNA in a patient’s blood sample, for example, which would help researchers understand how cancer mutates over time. For public health, it could help researchers monitor the frequency of antibiotic-resistant bacteria in a population. The scientific possibilities get very exciting very quickly,” Collins said in a press release.
Perhaps the most interesting applications of SHERLOCK are yet to come – and might occur outside the lab. In their article, the authors note that all the SHERLOCK reaction reagents can actually be lyophilized for long-term storage and then reconstituted on paper for applications at remote sites, at a projected cost of as low as $0.61 per assay.
“There is great excitement around this system,” said Deb Hung, a co-author from the Broad Institute Infectious Disease and Microbiome Program, in a press release. “There is still much work to be done, but if SHERLOCK can be developed to its full potential it could fundamentally change the diagnosis of common and emerging infectious diseases.”