Adding color to a miniature monochrome world
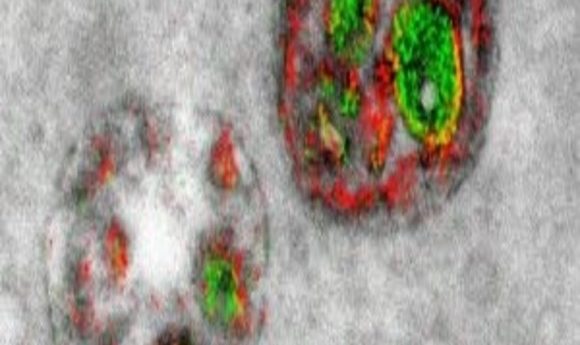
After 15 years of research, the late, Nobel Prize-winning, scientist Roger Tsien and his colleagues bring multicolor labels to electron microscopy. Three new discoveries have already emerged.
Every year over Christmas break, Roger Tsien gave himself a treat: he spent a few uninterrupted weeks in the lab working on chemistry projects he otherwise had little time for. The equipment was lightly used that time of year, and his phone and email were relatively silent. In 2003, his holiday project revolved around an idea he and his colleague Mark Ellisman had been mulling over for some time: adding dashes of color to the bleak black-and-white landscape of an electron micrograph.
Tsien pioneered colorful fluorescence in light microscopy, exploiting and developing multiple colors from green fluorescent protein (GFP) and designing other fluorescent proteins that highlight specific proteins and report signals under light microscopes. He won the Nobel Prize for Chemistry for that work in 2008. However, a similar technique to colorfully label electron micrographs, with their high resolution and fine details, proved elusive. Tsien and Ellisman wondered if using the rare earth metals known as lanthanides might help.
To see subcellular objects in a standard electron micrograph, researchers stain tissue with heavy metals, such as osmium, lead, or uranium, that adhere to, say, lipids or proteins and provide contrast. These metals non-selectively highlight membranes and other structures, delineating all of the organelles and most of the cytoskeleton within a cell. But pinpointing the exact location of something more specific, such as a particular protein, is difficult, and labeling more than one subcellular component is challenging for researchers.
“Electron microscopy has an advantage over light microscopy in that you get to see almost everything,” said Ellisman. “But you don’t necessarily have any localizing labels on all examples of a specific molecule.”
There have been attempts: Researchers linked gold to antibodies, but they don’t penetrate fixed tissue, or even thin sections, well. In 1994, Tsien and Ellisman added diaminobenzidine (DAB) to a cell and then oxidized it with photosensitizing dyes attached to a localizing agent such as an antibody or ligand, which generates a precipitate specific to a particular subcellular component (1). This negatively stains (surrounds) the target protein, though it’s still in black or white, which makes it hard to distinguish from surrounding monochromatic electron microscopy (EM) features.
Thus, the hope of labeling multiple different components of a cell with a palette of colors has remained a fantasy these long 13 years since Tsien began working in earnest with lanthanides during that Christmas vacation. Now, however, a new paper in Cell Chemical Biology (2) reports the first steps toward a technicolor EM world: two clear, crisp, red and green labels pseudocoloring a series of elegant electron micrographs.
Painting with Metals
When ringing phones and pinging emails pulled Tsien back into his office in early 2004, he handed the project over to senior scientist and chemist Stephen Adams. Tsien, Adams, and Ellisman have been working ever since to somehow “paint” various subcellular components, such as proteins or organelles, with distinguishable markers.
The researchers found that linking one lanthanide, such as cerium, to a DAB-like reagent and depositing the metal on a particular protein using the DAB photooxidation method (activation at a particular wavelength leading to precipitation) seemed to work. Then, using another lanthanide, such as praseodymium, and linking it to a second DAB-like chemical, they deposited this metal on another subcellular component using photosensitizers at a different wavelength that doesn’t excite the first DAB complex. (They could also do this with small-molecule probes or peroxidases). They then reacted and stained the sample with the normal osmium tetroxide-based heavy-metal staining. “The chemistry happens step-by-step, kind of like putting a leotard on a molecule,” said Ellisman.
They then used electron energy-loss spectroscopy (EELS) to separate the two lanthanides spectrally, implemented by energy-filtered transmission electron microscopy (EFTEM), and layered micrographs of the spatial distribution of each lanthanide on top of a conventional electron micrograph.
“We color-code the cerium and praseodymium images in whatever colors we would like to distinguish it from the gray background of the conventional image as we overlay it on top of that,” said Adams. In their proof-of-principle study, they clearly saw pseudo-colored green Golgi and red plasma membranes.
Next, they applied the technique to three problems that light microscopy had yet to solve. “Publishing papers on techniques is much more useful to the trainees and to the community if we demonstrate the techniques in the context of something that was otherwise hard or impossible to do,” said Ellisman. “We always challenge our group members to come up with, what’s the biological question?”
Ellisman’s lab studies astrocytes, so using the technique, they found that processes from two hippocampal astrocytes can share one synapse, which had not yet been determined since the resolution on light microscopes wasn’t high enough to see the fine processes of the astrocytes where they might touch synaptic terminals. In a second application, they saw that polyarginine-based cell-penetrating peptides enter cells by endocytosis, which was hypothesized, but not confirmed before.
“The cell-penetrating peptide story is part of a larger project in Roger’s lab on delivery for cancer detection, which uses these cell-penetrating peptides, so the ultimate fate of those in cells was of interest.” said Adams. “It’s a good example of where you might need an electron microscope to see where these are inside the vesicle, whether they’re on the inside or the outside.”
Lastly, they found that a newly-synthesized kinase, PKMzeta, in cultured neurons preferentially localizes to the post-synaptic membrane. “This came from an offshoot of other projects in the lab, where we were looking at where memories are stored in the brain,” said Adams. “This PKMzeta was a potential mechanism that had been proposed for involvement in memory storage and we were looking at its localization in neurons.”
Color Copying
Can other labs replicate this technique? It’s fiddly, said Adams. Working with the National Center for Microscopy and Imaging Research, of which Ellisman is Director, can help. “They’ve got expert hands to guide them throughout the process,” said Adams. “That will help because it’s very hard to go from a written procedure and then go into the lab and do it.”
Sadly, Tsien passed away unexpectedly in August 2016 without seeing this work published. He was only 64. Adams and other senior scientists have taken over his grants, and the Tsien lab continues, though some funding has disappeared with his death, like his HHMI grant. “We have to cut down substantially in the size of the lab, but still there are many projects we are busily working on,” said Adams.
And now that they have the method, “there’s a long queue for using it in experiments beyond the ones that we indicated in the paper,” added Ellison.
This won’t be Tsien’s last paper; there are additional publications in the works that will bear his name, continuing to make the micro world a more colorful place.