DNA loop extrusion: imagining to imaging
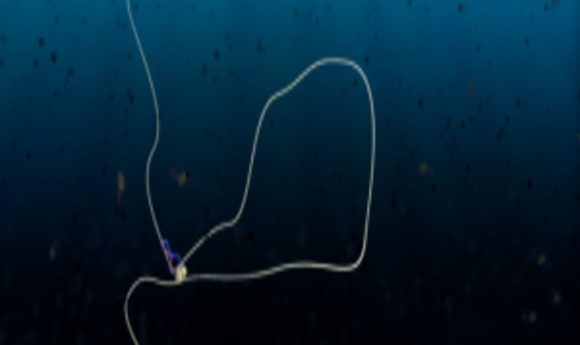
Researchers provide direct evidence of the DNA loop extrusion process for the first time using single molecule imaging.
A condensin protein complex creates a loop in DNA
Credit: Cees Dekker, TU Delft/Scixel
How does a meter-long strand of DNA fit inside a cell without forming a tangled mess? This accomplishment comes courtesy of structural maintenance of chromosomes (SMC) proteins, which orchestrate the formation of DNA loops and organize the genome architecture. The exact mechanism of DNA condensation by SMC proteins, such as condensin and cohesin has been a topic of debate for decades among researchers; some hypothesized that random crosslinks pull distal DNA sections together, while others proposed that the DNA extrudes through these ring-shaped proteins.
Now, Cees Dekker and his team at Delft University have settled this long-standing controversy by directly visualizing the DNA-condensin interaction at a single molecule level. Their findings, published today in Science, conclusively show condensin extruding DNA in real-time [1].
“It’s terrific; it’s wonderful news!” said Erez Lieberman Aiden from the Baylor College of Medicine, a leading researcher in genome folding who was not involved with this study. “This is very, very direct evidence that the extrusion model is in fact the correct model.”
Dekker has been intrigued by the interaction of SMC proteins with DNA for quite some time, but imaging labeled supercoiled DNA is not easy since the DNA nicks within seconds. Their recent success stemmed from the perfect combination of “right place, right time, right technique,” according to Mahipal Ganji, first author of this study.
Ganji developed an assay to image supercoiled DNA over a prolonged period using a transient and minimally damaging intercalating dye [2]. Armed with Dekker’s experience, coupled with the availability of purified active condensin from their collaborators at EMBL Heidelberg, he embarked upon the mission to resolve the role of condensin in DNA condensation.
In his experiments, Ganji anchored both ends of DNA to the surface of a microfluidic channel, ensuring that the DNA was slack enough to allow loop formation. When Ganji flushed in condensin and ATP in the channel, he observed the condensin reel in the DNA and form a loop that gradually increased in size.
“In terms of microscopy, it’s a real step forward to be able to see the dynamics of DNA enzymology in this kind of way,” said Lieberman Aiden.
From the initial experiments, the team noted an interesting observation: the extrusion process was asymmetric i.e. DNA was pulled in from one side as the condensin motor translocated, while the length of the other side remained constant.
“All models so far had assumed symmetric loop extrusion,” said Dekker, adding that this unexpected finding hinted at the underlying mechanism of condensin. The researchers suspected that one subunit of condensin functions like the clamp of a safety belt, firmly anchoring one side of the DNA. Ganji confirmed their hypothesis by demonstrating that the DNA slipped when he used a condensin mutant that could not fasten the belt in the experiments.
In the future, Dekker plans to test how other SMC proteins influence chromosomal arrangement, and then increase the complexity of the system by adding nucleosomes. Ultimately, he hopes to combine different in vitro processes to build a synthetic cell. “The bottom up approach is very powerful. We will see how far we can stretch this into living systems,” said Dekker.