This is what hibernation looks like at the molecular level
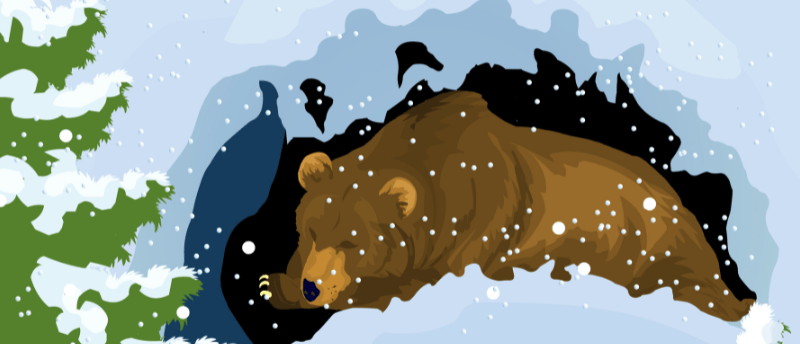
The molecular mechanisms underlying hibernation have been partially revealed thanks to skeletal muscle samples and the Mant-ATP chase assay.
Scientists have long studied hibernation; however, the molecular and cellular mechanisms underlying the dormant state have been neglected. An international collaboration led by researchers from the University of Copenhagen (Denmark) have investigated the molecular basis of hibernation, shedding light on the roles that myosin and metabolism play in regulating the body in this state.
Hibernation is a survival state that mammals of varying sizes enter during the winter months. This state is characterized by deep dormancy and significant reductions in metabolic activity, body temperature, respiration and heart rate. During this period, animals rely on the fat stores they’ve built up over the previous months to sustain their bodily functions. The state that the larger mammals enter is one of decreased metabolic rate, which allows them to conserve energy and remain alive during months of harsh weather and food scarcity. Whereas, smaller mammals that ‘hibernate’ actually enter a hypo-metabolic state termed ‘torpor’ and will experience periods where they temporarily raise their body temperature (interbout euthermic arousals; IBA).
Skeletal muscle comprises approximately 50% of mammals’ body mass and therefore must play a significant role in how an animal uses its energy and produces heat. “Until recently, energy consumption in skeletal muscles was thought to be primarily linked to the activity of myosin, which is involved in muscle contraction. However, there is growing evidence that, even when they are relaxed, skeletal muscles still use a small amount of energy,” explained lead author Christopher Lewis (University of Copenhagen).
Revealing the secrets behind one of nature’s greatest party tricks: stolonization
The mechanism of stolonization in Japanese green syllid worms has been revealed.
The team therefore wanted to investigate how energy consumption and myosin states were affected in hibernating muscles. There are two resting states – ‘disordered-relaxed’ (DRX) and ‘super-relaxed’ (SRX) – that myosin heads can be in, which determine how fast these motor proteins use up ATP.
The team took skeletal muscle samples from American black and brown bears to conduct their investigation in large mammals. First, they compared how myosin states and their energy consumption differed in active periods and hibernation, finding no significant difference in myosin-phase distribution across the active or hibernating periods. They then used a Mant-ATP chase assay to compare myosin’s ATP consumption in different activity states, again finding no significant difference in the energy consumption rates of myosin, which could protect mammals from muscle wastage during hibernation.
In smaller mammals, the team conducted similar experiments; however, due to small hibernating mammals having a slightly different hibernation experience – torpor and IBA – they found slightly different results. Although the proportion of myosin heads in DRX and SRX was similar across torpor, IBA and active state, they observed an overall increase in ATP consumption in the hibernating states. Due to smaller animals undergoing larger temperature fluctuations during hibernation, the team conducted another Mant-ATP chase assay in colder temperatures, revealing that ATP consumption increased in the IBA and active state, potentially indicating that non-shivering thermogenesis takes place in these states. However, they didn’t observe any cold-induced changes in myosin’s ATP consumption in the torpor state, indicating its more significant metabolic shutdown.
The study also evaluated protein changes in small hibernators, revealing that one myosin protein’s structure was affected by hibernation; the protein Myh2 was extensively phosphorylated during torpor, not during the other states. Myosin protein structure was not found to differ in active and hibernating states in bigger hibernators and therefore suggests that Myh2 hyper-phosphorylation is associated with torpor, rather than hibernation in general.
“Altogether, our findings suggest that ATP turnover adaptations in DRX and SRX myosin states occur in small mammals like the Thirteen-lined ground squirrel during hibernation in cold environments. In contrast, larger mammals like the American black bear show no such changes, likely due to their stable body temperature during hibernation,” concluded senior author Julien Ochala (University of Copenhagen).
Further study is needed to validate and expand the results found in this paper, but it provides an interesting starting point for understanding the molecular mechanisms underlying hibernation in both small and large mammals.